Although cardiac electrostimulation was recognised centuries ago, the technology of implantable heart rhythm monitoring and therapeutic devices has only been established in the last few decades. Recent advances in such technology have led to simpler implantation techniques, greater patient convenience with smaller device sizes, extended battery longevity, increased device safety and reliability, and improved clinical outcomes.
Introduction
The basis of modern cardiac pacing can be traced to the early nineteenth century, which saw the first hesitant development of cardiac electrostimulation.1,2 The nineteenth century concluded with a flurry of publications3 confirming that the heart could be reliably stimulated both directly, in open-chest dogs and, indirectly, in humans, via large electrodes placed over the praecordial area and on the back. In 1869 external electrical energy applied to the praecordium of a patient with tachycardia resulted in a slowing and regularising of the rhythm, probably the first reported case of external cardioversion.4,5 1871 saw the first successful temporary pacemaker applied to a young girl during anaesthetic-induced arrest. These endeavours allowed the first review article, entitled ‘electric stimulation of the heart in man’, to be published in 1889.
However, it was the description by Adams and subsequently Stokes6,7 of syncope and occasionally sudden death, associated with slow pulse rate, which spurred interest and research into the correction of bradycardias and the avoidance of cardiac arrest. The first experimental pacemaker, consisting of an electrical source and a mechanical device to control impulse formation and rate, was developed in 1932 by Hyman.8 The current was conducted via a needle introduced percutaneously to the right atrium.
In 1952 Zoll showed that in emergency situations stimulating between a praecordial plate and an oesophageal electrode successfully captured the heart.9 Long-term pacing, however, was intolerable. These limitations laid the foundation for the first transvenous bipolar catheter by Furman in 1958,10 connected to the patient by an external power source.

Subsequent advances in electronic systems, miniaturisation and improved chemical batteries allowed implantation, by Larsson et al.11 of the first self-contained pacemaker connected to the heart via epicardial leads. This paved the way for subsequent advances in pacing (figure 1). It became clear that syncope due to slow heart rates could be prevented, that patients’ life expectancy could be extended and that the systems, although large and cumbersome, were well tolerated.
The following decades were notable in the development of a number of technological advances:
- better lead design and manufacture
- epicardial to endocardial systems
- simpler implantation techniques and avoidance of thoracotomies
- improved battery technology and more reliable components with reduced battery drain
- hermetically sealed units extended device longevity.
Interest developed in the means to mimic the natural heart rate, which led to the development of two basic advances that form the cornerstone of modern cardiac pacing; demand pacing: and programmability.
The ability to sense spontaneous cardiac depolarisation and to inhibit pacemaker output allowed units to work only when required, thereby extending battery life. Safety was also improved by avoiding pacing into the T wave. The appearance of integrated circuits and microprocessors gave the first opportunities of externally altering pacemaker function, allowing adaptation to more physiological conditions. The development of lithium-based batteries was a significant advance, both in longevity and convenience. Currently, lithium-iodide systems form the basic power supply of modern implantable devices.
The advent of implantable loop recorders (ILRs) has resulted in a major breakthrough in diagnostic cardiology. These are wireless small electronic devices, which can be implanted subcutaneously close to the heart in a minor surgical procedure. The battery lasts for up to two years and the devices can continuously monitor the electrical activity of the heart; store rhythm disturbances within set parameters; and can be triggered by patients to store events. An ILR-guided strategy was shown to be safe and efficacious in directing therapy in patients with unexplained syncope.12
The present
Bradyarrhythmias
The demand pacemaker remains the fundamental unit in use today, and programmability has enabled greater flexibility in long-term patients’ management. Programmable functions such as current output and rate hysteresis conserve battery energy and reduce the frequency of inappropriate pacing. Reduction in generator size and better ergonomics has reduced complications such as erosion. Improved circuitry has further reduced battery current drain, despite increasingly complex programmable functions.
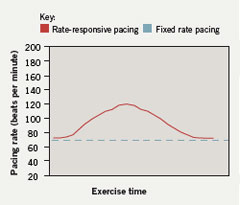
Sensors able to respond appropriately to patients’ physiological demands are now in standard use (figure 2). These use parameters such as:
- changes in pulmonary minute ventilation13
- motion detectors14 (piezo-electric crystals,
accelerometers) - an assessment of cardiac contractility15 (impedance, QT sensor)
- changes in central body temperature16
- venous oxygen saturation17
- muscular activity.18
Sensor output can be tailored to each individual patient’s needs using bi-directional programmability. Advances in lead technology include:
- bipolar electrodes
- better lead insulation
- smaller lead size
- improved lead tip technology (figure 3), for
example, laser-etched electrodes to increase
surface area.

Better passive and active fixation techniques have been developed and include steroid-eluting tips to reduce the acute tissue reaction. Better lead design has not always provided consistent results and some have proven less than satisfactory in long-term studies, producing lead fractures and rarely death.19 Continuous audit has shown remarkable lead longevity with less than an average replacement of 1–2%.20
Pacemakers capable of continuously determining lead impedance and threshold are now commercially available. Following lead fracture, automatic conversion from bi-polar to uni-polar mode occurs. Continuous interrogation of lead threshold (auto-capture) ensures that the minimal amount of current is provided for effective pacemaker capture, thereby extending battery life (SMART pacemakers).21
The recognition that ventricular pacing alone does not mimic normal physiological cardiac function led to the first atrial synchronous ventricular pacing in 1963 (Nathan et al.),22 followed by atrioventricular (AV) sequential pacemakers and finally true dual chamber systems. Initial problems related to atrial lead design and manufacture, complex programming problems, ventriculo-atrial conduction and pacemaker mediated-tachycardia have been largely overcome in recent years, mainly by improved programmability.
The major initial and perceived limitation with dual-mode, dual-pacing, dual-sensing (DDD) systems related to atrial arrhythmias. Tracking of the atrial tachycardia caused symptomatic and unwanted increase in the ventricular pace rates. Current DDD pacemakers can reliably detect atrial arrhythmias. Detection criteria, such as atrial rate and duration of tachycardia, are programmable. Automatic switching to a more appropriate mode (automatic mode switching) avoids unnecessary ventricular tracking. In patients with intermittent AV block, rate sensor back-up can also be provided. Many standard DDD pacemakers switch to rate sensing ventricular demand pacing (ventricular-pacing, ventricular-sensing, inhibited rate responsive [VVIR] or dual chamber-pacing, dual chamber-sensing, inhibited rate responsive [DDIR]) during atrial fibrillation and switch back to DDD mode when sinus rhythm spontaneously restores.
DDD systems have some inherent limitations where intrinsic sinus node function is impaired but the addition of a biosensor restores the system’s capabilities to as near physiological as possible. Troublesome atrial arrhythmias can also be effectively dealt with and the presence of paroxysmal atrial fibrillation is no longer the contraindication to dual chamber pacing it once was.
The development of physiological pacing systems heralded a leap forward in technology but no comparative clinical trials had been done until recently. Comparison of pacing modes, non-physiological versus physiological, has been studied recently with conflicting trial results.23-25 Atrial pacing appeared to be the best option but in some studies there was little difference between ventricular-pacing, ventricular-sensing inhibited (VVI) and physiological systems. Care must be taken in interpreting those results as in some studies endpoints were measured only over relatively short periods of time. Data from large multi-centre studies are still awaited.
Tachyarrhythmias
Pacing can terminate both supraventricular and ventricular arrhythmias. Early anti-tachycardia pacing (ATP) used pacemakers that were activated with external magnets, usually initiating under-drive or burst pacing under medical supervision. Current implantable systems have automatic algorithms capable of reliably terminating supraventricular arrhythmias,26,27 although these have been largely superseded by radiofrequency ablation. However, new devices such as the AT500 pacemaker are successfully being used to treat atrial tachycardias that fail to respond to ablation therapy.
ATP can also be used to terminate ventricular arrhythmias.28 Acceleration of the arrhythmia or degeneration to ventricular fibrillation limited widespread use but the development of the implantable defibrillator (ICD), first described by Mirowski,29 has revolutionised the management of such patients. At present, ICD detection of arrhythmias is based upon changes in heart rate.
In the late 1980s and early 1990s, ICDs could not differentiate between ventricular and supra-ventricular tachycardia. The addition of more complex algorithms and sensing from an atrial lead has solved many of these diagnostic problems. One advantage has been avoiding unnecessary and inappropriate shocks, although there still remain situations where shocks can be delivered inappropriately. In single-lead systems, increasingly complex detection algorithms have been used to overcome the lack of an atrial lead. These include sudden onset of the arrhythmia and stability of its rate, the theory being that most pathological tachycardias start suddenly as opposed to sinus tachycardia, which tends not to; rate stability is aimed at the detection of atrial fibrillation.30 In addition, some devices measure QRS width, which tends to increase dramatically during ventricular tachycardia compared with sinus rhythm.31
Although successful in the main, the addition of many of these parameters significantly reduces sensitivity and specificity of tachycardia detection and may lead the device to miss important arrhythmias. Dual chamber ICDs have better diagnostic capabilities, making inappropriate shocks during atrial arrhythmias infrequent. Improvement in memory storage and in the recording of high-grade cardiac electrograms has extended their diagnostic capabilities and allows physicians to check on the device therapy history.
Current ICDs are becoming more widespread in their use. The generator size can be as small as 30 cm2 for single-lead systems. The recognition that biphasic shocks were more effective than monophasic shocks, and the development of the ‘hot can’ whereby the generator is active (the anode), has improved shock efficiency and reduced battery drain. Current ICDs, delivering one shock per month, with 100% back-up pacing, may endure 5–7 years before battery depletion.
ICD technology is now comparable to state of the art pacing systems. Small generator sizes, biosensors, increased longevity, DDD systems and pectoral implants allow ease of use and increased implantation rate. Recent multi-centre studies such as the Multicenter Automatic Defibrillator Implantation Trial (MADIT 1&2),32 Comparison of Medical Therapy, Pacing and Defibrillation in Heart Failure (COMPANION),33 and Sudden Cardiac Death in Heart Failure Trial (SCD-HeFT),34 have confirmed the superiority of implantable defibrillators in some patients over chronic drug therapy and have identified high-risk patients who would benefit from implantation of ICDs over and above long-term drug therapy.
The National Institute for Health and Clinical Excellence (NICE) has recently revised the indications for ICD implantation. New guidance was issued in January 2006 (table 1).35

The future
Heart failure
With developing technologies the accepted indications for implantable cardiac devices are currently being extended. Heart failure is receiving intense interest. Where left ventricular function is impaired with evidence of dyskinesia, contraction can be ‘resynchronised’ by pacing the left and right ventricles simultaneously, improving overall cardiac output. Bi-ventricular pacing to resynchronise ventricular activation (cardiac resynchronisation therapy [CRT]) is now possible by introducing an additional left ventricular pacing electrode via the coronary sinus (CS).36
Indications for CRT include broad QRS complexes (115–130 milliseconds or greater) and left ventricular dysfunction and dilatation with New York Heart Association (NYHA) class III–IV. CRT has provided a beneficial haemodynamic effect in some patients, with clear evidence of symptomatic improvement shown on initial clinical studies. Recently published results from Cardiac Resynchronization-Heart Failure (CARE-HF) study have provided new evidence that bi-ventricular pacing reduces both morbidity and mortality.37,38
Cardiomyopathy
Dilated cardiomyopathy is an increasingly recognised consequence of long-term right ventricular apical pacing. Measures such as atrial-pacing, atrial-sensing inhibited (AAI) pacing, right ventricular septal pacing, and extension of AV delay in DDD systems to allow for intrinsic ventricular activation were used to avoid right ventricular apical pacing. Upgrading to bi-ventricular pacing systems appears to improve left ventricular function in affected patients with variable success rates.39 Advances in deflectable catheters that deploy CS leads of bi-ventricular pacemakers have significantly reduced procedure times. Pre-shaped CS lead tips have allowed quicker positioning and hence simpler implantation of CRT devices.
Atrial fibrillation
Various approaches have been used to attempt pacing for the prevention of atrial fibrillation. Studies have demonstrated an electrical delay within the atrium in some patients with paroxysmal atrial fibrillation.40 Theoretically, shortening this delay and altering the effect of atrial refractory periods may reduce the frequency of paroxysms. Dual site atrial pacing, either at the high right atrium and coronary sinus or at the high right atrium and intra-atrial septum, has been attempted with mixed results,41,42 and it is difficult to identify those patients who obtain the greatest effects.
Currently, atrial fibrillation preventative algorithms are being tested. These use single site pacing (usually from the high right atrium) and include prevention of sinus bradycardia–related arrhythmia by a continuous over-drive atrial pacing, or the prevention of post atrial ectopic delay. Results from large patient groups are awaited and the effect of both bi-atrial pacing in combination with newer algorithms remains to be proven.
Remote programming
Trans-telephonic interrogation of pacemakers is already possible, as is wireless interrogation of ICDs. Developments in global positioning systems (GPS) may allow remote interrogation and programming of pacemakers wherever patients are on the planet. These developments are ideal in rural areas, but their safety, particularly for reprogramming ICDs at a distance, remains to be proven.
Conclusion
From the initial demonstration that the heart could be stimulated by external electrical energy, technological developments over the past 50 years have confirmed reliable and effective cardiac pacing. A reduction in generator size, improved lead reliability, improved batteries and longevity, and the development of implantable defibrillators have extended device therapy for many patients.
Multi-site pacing in heart failure and anti-tachycardia algorithms for atrial fibrillation are heralding a resurgence of interest in pacing and the superiority of device therapy over chronic drug therapy is now well established. Device therapy is and will continue to remain the mainstay treatment for patients with both tachy- and bradyarrhythmias.
Conflict of interest
None declared.
Key messages
- Current technology of implantable cardiac devices allows accurate diagnosis of rhythm disorders and is capable of delivering effective therapy for both tachy- and bradyarrhythmias
- Transvenous pacing leads and lithium batteries have greatly influenced implantation simplicity and unit longevity, respectively
- The advent of telemetric programmability and demand pacing has enhanced both device reliability and patients’ convenience. Cardiac resynchronisation therapy has recently been shown to improve mortality and morbidity in heart failure patients
- Future developments in global positioning systems (GPS) may allow remote interrogation and programming of devices, thereby adding to patients’ convenience and reducing the workload on pacing clinics
References
- Harken DE. Pacemakers, past-makers, and the paced: an informal history from A to Z (Aldini to Zoll). Biomed Instrum Technol 1991;25:299–321. Erratum in: Biomed Instrum Technol 1991;25:456.
- Fontaine G. History of electricity in cardiology. Arch Mal Coeur Vaiss 1988;81:1421–6.
- Bouyrain Y. [Cardiologic therapeutics in the 19th century]. Ann Cardiol Angeiol (Paris) 1983;32:
79–82. - Bloomfield DK. Early work on external electrical defibrillation. N Engl J Med 1980;303:1366–7.
- Luderitz B. History of cardiac rhythm disorders. Z Kardiol 2002;91(suppl 4):50–5.
- Adams R. Cases of diseases of the heart, accompanied with pathological observations. Dublin Hospital Reports 1827;4:353–453.
- Stokes W. Observations on some cases of permanently slow pulse. Dublin Quarterly Journal of Medical Science 1846;2:73–85. Reprinted in: Medical Classics 1939;3:727–38.
- Senning A. Cardiac pacing in retrospect. Am J Surg 1983; 145:733–9.
- Madsen JK, Meibom J, Videbak R, Pedersen F, Grande P. Transcutaneous pacing: experience with the Zoll noninvasive temporary pacemaker. Am Heart J 1988;116(1 Pt 1):7–10.
- Furman S, Robinson G. The use of an intracardiac pacemaker in the correction of total heart block. Surg Forum 1958;9:245–8.
- Larsson B, Elmqvist H, Ryden L, Schuller H. Lessons from the first patient with an implanted pacemaker: 1958-2001. Pacing Clin Electrophysiol 2003;26(1 Pt 1):114–24.
- Brignole M, Sutton R, Menozzi C et al.; International Study on Syncope of Uncertain Etiology 2 (ISSUE 2) Group. Early application of an implantable loop recorder allows effective specific therapy in patients with recurrent suspected neurally mediated syncope. Eur Heart J 2006;27:1085–92.
- Duru F, Cho Y, Wilkoff BL et al. Rate responsive pacing using transthoracic impedance minute ventilation sensors: a multicenter study on calibration stability. Pacing Clin Electrophysiol 2002;25:1679–84.
- Lau CP, Stott JR, Toff WD, Zetlein MB, Ward DE, Camm AJ. Selective vibration sensing: a new concept for activity-sensing rate-responsive pacing. Pacing Clin Electrophysiol 1988;11:1299–309.
- Arthur W, Kaye GC. Clinical use of intracardiac impedance: current applications and future perspectives. Pacing Clin Electrophysiol 2001; 24(4 Pt 1):500–06.
- Novak M, Smola M, Psenicka M, Stanek F. Is the response to cardiac pacing controlled by central venous temperature physiological? Bratisl Lek Listy 1997;98:613–15.
- Rodriguez Garcia J, Silvestre Garcia J, Coma Samartin R et al. Biosensors and rate-responsive cardiac pacing. Rev Esp Cardiol 1990;43(suppl 2):
58–66. - Morton PG. Rate-responsive cardiac pacemakers. AACN Clin Issues Crit Care Nurs 1991;2:140–9.
- Kawanishi DT, Brinker JA, Reeves R et al. Kaplan-Meier analysis of freedom from extraction or death in patients with an Accufix J retention wire atrial permanent pacemaker lead: a potential management tool. Pacing Clin Electrophysiol 1998;21
(11 Pt 2):2318–21. - Deharo JC, Djiane P. Pacemaker longevity. Replacement of the device. Ann Cardiol Angeiol (Paris) 2005;54:26–31.
- Saoudi N, Appl U, Anselme F et al. How smart should pacemakers be? Am J Cardiol 1999;83(5B):180D–186D.
- Nathan DA, Center S, Wu CY, Keller W. An implantable synchronous pacemaker for the long term correction of complete heart block. Am J Cardiol 1963;11:362–7.
- Fananapazir L, Srinivas V, Bennett DH. Comparison of resting hemodynamic indices and exercise performance during atrial synchronized and asynchronous ventricular pacing. Pacing Clin Electrophysiol 1983;6(2 Pt 1):202–09.
- Huang M, Krahn AD, Yee R, Klein GJ, Skanes AC. Optimal pacing for symptomatic AV block: a comparison of VDD and DDD pacing. Pacing Clin Electrophysiol 2003;26:2230–4.
- Huang M, Krahn AD, Yee R, Klein GJ, Skanes AC. Optimal pacing for symptomatic AV block: a comparison of VDD and DDD pacing. Pacing Clin Electrophysiol 2004;27:19–23.
- Bruce GK, Friedman PA. Device-based therapies for atrial fibrillation. Curr Treat Options Cardiovasc Med 2005;7:359–70.
- Baszko A, Rinaldi CA, Simon RD, Gill JS. Atrial fibrillation current and future treatments: radiofrequency ablation and novel pacing techniques. Int J Clin Pract 2002;56:370–6.
- Weber M, Block M, Bansch D et al. Antitachycardia pacing for rapid VT during ICD charging: a method to prevent ICD shocks. Pacing Clin Electrophysiol 2001;24:345–51.
- Janse MJ. A brief history of sudden cardiac death and its therapy. Pharmacol Ther 2003;100:89–99.
- Mletzko R, Anselme F, Klug D. Enhanced specificity of a dual chamber ICD arrhythmia detection algorithm by rate stability criteria. Pacing Clin Electrophysiol 2004;27:1113–19.
- Klingenheben T, Sticherling C, Skupin M, Hohnloser SH. Intracardiac QRS electrogram width-an arrhythmia detection feature for implantable cardioverter defibrillators: exercise induced variation as a base for device programming. Pacing Clin Electrophysiol 1998;21:1609–17.
- Zwanziger J, Hall WJ, Dick AW et al. The cost effectiveness of implantable cardioverter-defibrillators: results from the Multicenter Automatic Defibrillator Implantation Trial (MADIT)-II. J Am Coll Cardiol 2006;47:2310–18.
- Salukhe TV, Dimopoulos K, Francis D. Cardiac resynchronisation may reduce all-cause mortality: meta-analysis of preliminary COMPANION data with CONTAK-CD, InSync ICD, MIRACLE and MUSTIC. Int J Cardiol 2004;93:101–03.
- Borggrefe M, Wolpert C. Amiodarone or ICD in patients with cardiac insufficiency. SCD-HeFT Study. Internist (Berl) 2006;47:89–91.
- National Institute of Clinical Excellence. TA95 Arrhythmia – implantable cardioverter defibrillators (ICDs): Guidance (Word). Available from: http://www.nice.org.uk/page.aspx?o=TA095guidanceword
- Seidl K, Rameken M, Vater M, Senges J. Cardiac resynchronization therapy in patients with chronic heart failure: pathophysiology and current experience. Am J Cardiovasc Drugs 2002;2:219–26.
- Calvert MJ, Freemantle N, Yao G et al. Cost-effectiveness of cardiac resynchronization therapy: results from the CARE-HF trial. Eur Heart J 2005;26:2681–8.
- Cleland JG, Coletta AP, Lammiman M et al. Clinical trials update from the European Society of Cardiology meeting 2005: CARE-HF extension study, ESSENTIAL, CIBIS-III, S-ICD, ISSUE-2, STRIDE-2, SOFA, IMAGINE, PREAMI, SIRIUS-II and ACTIVE. Eur J Heart Fail 2005;7:1070–5.
- Dilaveris P, Pantazis A, Giannopoulos G, Synetos A, Gialafos J, Stefanadis C. Upgrade to biventricular pacing in patients with pacing-induced heart failure: can resynchronization do the trick? Europace 2006;8:352–7.
- Dabrowska-Kugacka A, Lewicka-Nowak E, Kutarski A et al. Hemodynamic effects of alternative atrial pacing sites in patients with paroxysmal atrial fibrillation. Pacing Clin Electrophysiol 2003;26
(1 Pt 2):278–83. - Bruce GK, Friedman PA. Device-based therapies for atrial fibrillation. Curr Treat Options Cardiovasc Med 2005;7:359–70.
- Prakash A. Pacing for the prevention of atrial fibrillation. Curr Opin Cardiol 2002;17:73–81.