Cerebrogenic control of cardiac function is well recognised and acute neurological events, including epileptic seizures, may cause a disturbance of cardiac function even in the absence of significant cardiac structural or electrophysiological abnormalities. Sudden unexpected death in epilepsy (SUDEP) is a major cause of mortality in patients with epilepsy. Cardiac dysrhythmias are a potential cause of SUDEP.
Patients with epilepsy may be predisposed to developing arrhythmias due to a number of factors including chronic autonomic dysfunction, effects of anti-epileptic medication and a common genetic susceptibility. Future work should include the evaluation of inter-ictal and ictal electrophysiological, cardiorespiratory and metabolic variables in a large population of patients, including in specific syndromes, to further establish the pathophysiological mechanisms of SUDEP. A key aim is to stratify the risk of SUDEP for an individual patient and, ideally, identify potential therapeutic targets.
Introduction
Anatomical and functional connections between the brain and heart in both health and disease have long been established. Cardiac arrhythmias and ST segment changes have been observed with acute intracerebral events such as sub-arachnoid haemorrhage or cerebrovascular accidents1 and, more recently, the interaction of the heart and brain in patients with epilepsy has been the subject of intense scrutiny. This has been driven by the publication of a number of important studies that have documented the frequent occurrence of cardiac rhythm changes during epileptic seizures,2,3 and the lack of a clear pathophysiological mechanism for sudden unexpected death in epilepsy (SUDEP), an unexplained cause of death for over 500 patients with epilepsy in the UK each year. The study by Chaila and colleagues lends support to the now fairly well-established thesis that disturbed cerebral electrical activity during an epileptic seizure may cause transient cardiac arrhythmias (see pages 245–8). It is important to note, however, that although small series observational studies are helpful in raising awareness of this poorly understood entity, unfortunately, they have little capacity to inform the debate about possible mechanisms, risk factors and preventative measures.
There has always been a degree of clinical overlap between cardiology and neurology, and, more specifically, epileptology. Patients with episodes of collapse are frequently referred to either cardiologic or neurologic services and the correct diagnosis is often elusive. Misdiagnosis is common and possibly affects up to 20 to 30% of adults with a diagnosis of epilepsy.4,5 For example, when 74 patients previously diagnosed with epilepsy were investigated with tilt-table testing, prolonged electrocardiogram (ECG) monitoring, blood pressure and ECG-monitored carotid sinus massage, an alternative cardiologic diagnosis was found in 31 (41.9%) of patients, including 13 taking anti-epileptic medication.6 In addition to an isolated cardiologic or epileptic basis for an episode of collapse, there exists the phenomenon of cerebrogenic cardiac arrhythmias, which further confounds the diagnostic process (figure 1).
”]![Br-J-Cardiol-2010-17-224-fig-1 Figure 1. Standard electroencephalograph (EEG) recording from a patient with focal epilepsy and recurrent seizures characterised by sudden onset of déjà vu, a rising epigastric sensation followed by loss of awareness and then collapse with marked facial pallor. The EEG shows right-sided rhythmic epileptic activity (most easily seen in the RsSph electrode trace) followed several seconds later by a 10–12 second period of cardiac asystole (electrocardiogram [ECG] trace – bottom red line). Anti-epileptic medication was commenced and a permanent pacemaker was fitted and she has remained well with occasional déjà vu but no episodes of collapse or loss of consciousness](https://bjcardio.co.uk/files/uploads/2010/09/Br-J-Cardiol-2010-17-224-fig-13.png)
Ictal arrhythmias
It is acknowledged that the presence of ECG changes, for example T wave inversion, ST segment elevation and a prolonged QT interval, in patients with intracranial pathology, such as subarachnoid haemorrhage, are a manifestation of massive catecholamine release and autonomic dysregulation resulting in ventricular wall motion abnormalities, vasospasm and subsequent cardiac contraction band necrosis.7 Predominantly neurogenic, rather than humorally driven, autonomic dysfunction has been postulated as the cause of ECG abnormalities during epileptic seizures.
Arrhythmias, conduction block and repolarisation ECG abnormalities, such as atrial fibrillation, marked sinus arrhythmia, supraventricular tachycardia, atrial and ventricular premature depolarisation, bundle-branch block, high grade atrioventricular conduction block, ST segment depression and T wave inversion, have been reported in up to 56% of seizures and are more common in nocturnal, prolonged and generalised seizures, rather than in focal seizures or those occurring during wakefulness.8,9 Sinus rate change is the most common cardiac accompaniment to ictal discharge with sinus tachycardia reported in 50–100% of seizures.2,3,10 Although the heart rate in ictal tachycardia is typically 100–120 beats per minute,3 there are reports of rates exceeding 170 beats per minute, even during simple partial seizures.2,11 Ictal tachycardia is most commonly seen in the early ictal phase, soon after seizure onset,10-12 or rarely before clear evidence of electroclinical onset.13 This contrasts with ictal bradycardia, which is seen during the late ictal phase or in the immediate post-ictal period.14,15 There is some evidence for right-sided cerebral lateralisation and temporal lobe localisation in patients with ictal tachycardia,10,12,16 corroborating the reports of early experimental and clinical stimulation studies,17-19 although it is important to note that most temporal lobe seizures are associated with ictal tachycardia, irrespective of lateralisation. Most recently, a patient was observed to develop ventricular fibrillation requiring cardiopulmonary resuscitation, including defibrillation, during a secondarily generalised tonic-clonic seizure arising from the right temporal lobe. The patient recovered and subsequent cardiac investigations, including catheter angiography and cardiac electrophysiology, were normal.20
Although ictal tachycardia is almost universally observed, ictal bradycardia has received more attention due to the potential progression to cardiac asystole and intuitive but, as yet, unproven association with SUDEP. The first report of ictal asystole was by Russell in 1906, who noted the disappearance of a young male patient’s pulse during a seizure.21 The published literature since that time is, unsurprisingly, mostly case reports or small series studies, which significantly limit the number and confidence of any conclusions extracted from the data. Ictal bradycardia is observed in <5% of recorded seizures,2,12,22,23 but may occur in a higher percentage of patients, because a consistent cardiac response to each apparently electroclinically identical seizure is not seen.2
A recent literature review by Britton revealed that of 65 cases of ictal bradycardia with sufficient electroencephalograph (EEG) and ECG data, seizure onset was localised to the temporal lobe in 55%, the frontal lobe in 20%, the frontotemporal region in 23%, and the occipital lobe in 2%. Information regarding seizure-onset lateralisation was available in 56 cases. Seizure onset was lateralised to the left hemisphere in 63%, the right in 34%, and bilaterally in 4%.15 It appears, therefore, that there is a trend towards the left temporal lobe being implicated in ictal bradycardia, however, this is not sufficiently specific to be valuable in localising semiological information.15,23 Of greater interest, is the frequent observation of bilateral ictal activity during bradycardia.15,24,25 This may cause a more significant imbalance of parasympathetic and sympathetic dysfunction than unilateral stimulation via either cortico-cardiac pathways or through connections with subcortical and brain stem regions, which are frequently activated in seizures and which may potentially contribute to a bradycardic response.26,27
Ictal asystole, lasting between four and 60 seconds, is reported, albeit rarely, in patients with refractory epilepsy2,24,28 (figure 1). In addition, experimental data suggest that ictal bradyarrhythmias can lead to complete heart block.29 It is possible, however, that bradycardia and asystole are conditions with distinct pathophysiological bases rather than two points on a continuum of cerebrogenic arrhythmias. However, the characteristics of the epilepsy with respect to localisation, lateralisation, seizure types and population demographics are identical to those of patients with ictal bradycardia, suggesting that the two entities are possibly linked. Short periods of EEG/ECG monitoring may underestimate the prevalence of ictal asystole. For example, Schuele searched a database of 6,825 patients undergoing in-patient video-EEG monitoring and found ictal asystole in only 0.27% of all patients with epilepsy. In contrast, our group reported on 19 patients with refractory focal epilepsy who were implanted with an ECG loop recorder for up to 18 months. Over 220,000 patient hours of ECG recording were monitored, during which time 3,377 seizures (1,897 complex partial or secondarily generalised tonic-clonic seizures and 1,480 simple partial seizures) were reported by patients. Cardiac rhythm was captured on the implantable loop recorders in 377 seizures. Ictal bradycardia, defined as a rate of less than 40 beats per minute, was seen in 0.24% of all seizures over the study period, and 2.1% of the recorded seizures. Seven of the 19 patients experienced ictal bradycardia. Four of these had severe bradycardia or periods of asystole that led to the insertion of a permanent pacemaker. The small number of patients involved precluded statistical analysis of localisation and lateralisation data. There was no clear correlation between cardiac events and specific anti-epileptic drugs. Notably, only a small proportion of seizures for every patient were associated with significant cardiac events despite identical seizure characteristics.2 The wider significance of these findings remains to be established but may be addressed by a larger UK-based multi-centre study that is currently underway. The identification and targeting of patients at risk of ictal asystole, preferably with an inter-ictal surrogate marker, is an important goal.
Extrapolation of ictal bradyarrhythmias to a mechanistic explanation for SUDEP remains elusive. This is, at least partly, due to a lack of clinical evidence of common factors shared by patients with ictal bradyarrhythmias and SUDEP, and the difficulty in ascertaining the importance of ictal bradyarrhythmias in SUDEP in relation to other proposed mechanisms, including other intrinsic cardiac abnormalities or apnoea and hypoxia, which may aggravate arrhythmias.
It is possible that patients with epilepsy are predisposed to developing cardiac arrhythmias during seizures due to a number of factors. These include the chronic exposure to potentially destabilising anti-epileptic medications, which may have additional, adverse cardiac and autonomic effects, the possibility of a shared genetic susceptibility for epilepsy and cardiac channelopathies and the long-term effects of recurrent seizures on the heart leading to structural cardiac abnormalities or on the autonomic nervous system resulting in, for example, changes in heart rate variability (figure 2).
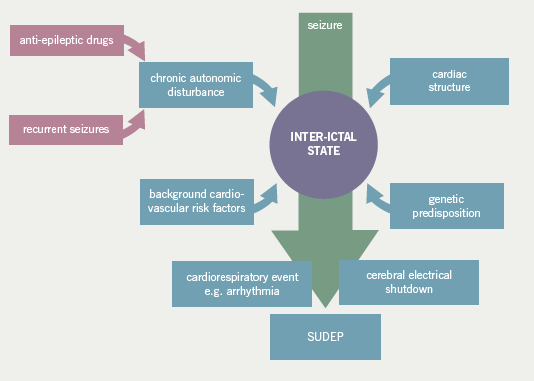
Cardiac pathology
Minor, non-specific pathological change, presumed to be non-fatal, such as atherosclerosis, conducting system fibrosis, diffuse myocardial fibrosis and myocyte vacuolisation, has been identified in a greater number of SUDEP cases compared with control cases in a number of studies.30-32 Interestingly, myocyte vacuolisation has also been observed in rats who developed asystole following stimulation of the insular cortex.32 Myocyte vacuolisation is considered to be a reversible pathological entity, occurring in the context of subendocardial ischaemia.33 However, the patients, universally, had normal appearing coronary arteries. It has been postulated that neurogenic coronary vasospasm may be implicated, and that, if recurrent, may eventually progress to perivascular and interstitial fibrosis.34 This may, in turn, predispose the heart to arrhythmogenesis, particularly in the setting of considerable autonomic imbalance during seizures.35,36 More recently, qualitative and quantitative histopathological assessments of myocardial fibrosis were undertaken in SUDEP and age- and gender-matched non-SUDEP cases. Although visual assessment showed significantly more fibrosis in the SUDEP cases, this was not verified on quantitation. Furthermore, no abnormalities of the cardiac conducting system were demonstrated.37
Overall, the full characterisation of the relationship between myocardial pathology and acute and recurrent seizures remains unclear at the present time. Future studies should utilise standardised histopathological qualitative and quantitative protocols with extensive tissue sampling, and correlate findings with clinical data to ensure accurate and reproducible data are obtained.
Genetic susceptibility
It is possible that specific epilepsy syndrome subtypes carry an increased risk of sudden death due to phenotypic expression in other cerebral, and possibly cardiac, structures. For example, Rett syndrome, typically due to mutations in the MECP2 gene, is associated with brain stem immaturity and autonomic, particularly respiratory, instability in association with epilepsy. In addition to respiratory abnormalities, which include apneustic breathing and hyperventilation, patients with Rett syndrome may also present with a prolonged QT interval and reduced heart rate variability.38 The co-existence of cardiac arrhythmia and central apnoea may act synergistically in the development of sudden death. Of recent interest in the pursuit for pathophysiological mechanisms of SUDEP is the potential association between cardiac and epileptic ion channelopathies.39 For example, it is well known that long-QT syndrome, a defect of cardiac repolarisation typically due to potassium or sodium channel mutations, produces prolongation of the action potential, propensity to malignant tachyarrhythmias and sudden death.40 Ion channelopathies are also implicated in familial, monogenic idiopathic epilepsies, such as generalised epilepsy with febrile seizures plus (GEFS+).41 A recent report of a GEFS+ pedigree with a SCN1A mutation and two cases of SUDEP is of interest in this respect, and severe myoclonic epilepsy in infancy (Dravet syndrome) is also associated with SCN1A mutations and patients have an increased risk of sudden death.42 It is noteworthy that SCN1A, although primarily expressed in the brain, can also be found in the rodent heart where it is involved in the pacemaker function of the sinoatrial node.43 Nevertheless, direct evidence linking these cardiac inherited gene determinants and SUDEP is lacking at present.
Inter-ictal cardiac and autonomic dysfunction
At the simplest level, inter-ictal cardiac function can be evaluated by visually assessing a standard 12-lead ECG, primarily for evidence of conduction abnormalities, although in patients with epilepsy these are frequently normal2,3,44 or show only minor, non-significant changes.45 However, a recent preliminary study of 128 patients with severe refractory epilepsy and learning disability, revealed inter-ictal ECG abnormalities in approximately 60% of patients, including first degree atrio-ventricular block and poor R wave progression.46 Potentially, a number of important confounding factors exist though, including whether this study population is adequately representative, whether account was made of the presence of systemic co-morbidity or use of psychotropic medication, and whether the conduction abnormalities are clinically significant.
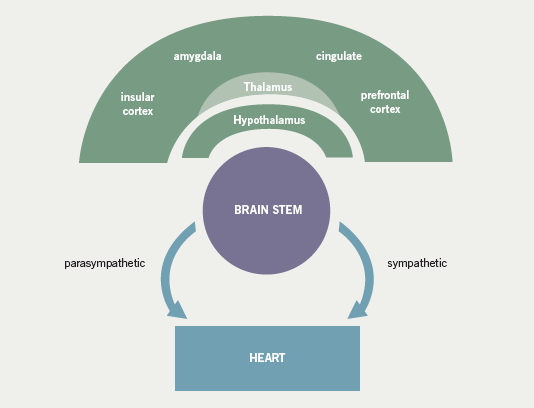
Early experimental studies demonstrated that inter-ictal epileptiform activity was associated with sympathetic and parasympathetic autonomic dysfunction, in a time-locked synchronised pattern29,47 (figure 3). In the first clinical reports, analysis of inter-ictal heart rate variability (HRV) in 19 patients with refractory temporal lobe epilepsy revealed frequent, high-amplitude, fluctuations in heart rate, which were most pronounced in patients with pharmacoresistant epilepsy who were deemed unsuitable for epilepsy surgery.48 Reduced sympathetic tone, demonstrated by decreased low frequency power, has been seen in both focal and, albeit less markedly, primary generalised epilepsy.44,48-50 It is important to note that reduced HRV has been reported in infants with aborted sudden infant death syndrome,51 in heart transplant patients52 and as an independent risk factor for sudden arrhythmic death after myocardial infarction.53 These findings have been corroborated by more recent studies,54-56 although the pattern of inter-ictal autonomic disturbance in patients with epilepsy is contentious. For example, Evrengul et al. reported an increase in low frequency power and a reduction of high frequency values consistent with an increase in the sympathetic control of the heart rate in patients with untreated generalised tonic-clonic seizures.57 It is possible that the disparity between the studies may be, at least partly, due to anti-epileptic medication. Despite a number of descriptive and controlled studies, no specific anti-epileptic drug has been clearly associated with an increased risk of SUDEP,30,58-63 although a small number of studies have implicated treatment with carbamazepine as an independent risk factor.64-66 For anti-epileptic medication in general, proposed mechanisms include perturbed HRV, lengthening of the QT interval on the ECG combined with a mild pro-arrhythmic effect of epileptic seizure discharges, or excessive post-seizure brain stem inhibition producing a blunting or transient abolition of the central hypoxic and hypercarbic respiratory drive, with consequent post-ictal respiratory arrest.64,66,67 Elevated serum levels of carbamazepine have been associated with an increased risk of SUDEP even after adjustments for seizure frequency have been made. Frequent drug changes and multiple concomitant anti-epileptic drugs, conventional markers of severe and unstable epilepsy, increased this risk synergistically.68 On this basis, it is difficult to know whether a high carbamazepine level is an independent risk factor or is merely representative of challenging epilepsy. Tomson showed that patients on carbamazepine had a significantly lower standard deviation of RR-intervals, low frequency power and a reduced frequency/high frequency power ratio than matched healthy control subjects. In patients on sodium valproate, only the ratio of low frequency/high frequency power was lower.49 Inter-ictal autonomic dysfunction associated with carbamazepine use has also been implicated in a number of similar studies. Isojarvi et al. evaluated 84 patients with a variety of epilepsies, and observed autonomic dysfunction in only those patients taking carbamazepine.69 Confusingly, rapid withdrawal of carbamazepine has been associated with both increased sympathetic tone during sleep as measured by the low-to-high frequency ratio66 and a significant reduction in HRV in both the time and frequency domains with, in particular, a significant reduction in low frequency power and sympathetic tone.70 The reason for the divergence between these studies is not clear, but it is important to note that both were small series studies and may have lacked sufficient power to convincingly demonstrate a clear effect. Furthermore, there may have been methodological differences. To address the possible modulatory effect of anti-epileptic medication, patients with untreated epilepsy have been studied and shown to have both increased low frequency power, and thus augmented sympathetic tone,57 and, in a similar study, normal HRV parameters.71 Again, it is difficult to satisfactorily explain the variance in the results, although important differences in the study population may be, at least partly, responsible.71 In an interesting study of patients with chronic temporal lobe epilepsy (TLE), post-ganglionic cardiac sympathetic innervation was quantified using [123I]metaiodobenzylguanidine-single photon computed tomography (MIBG-SPECT).
Cardiac MIBG uptake was significantly less in the TLE patients than in the controls, but did not differ between subgroups with and without carbamazepine treatment. The findings are consistent with either post-ganglionic trans-synaptic degeneration resulting from a prolonged increase in central sympathetic inter-ictal discharges or competitive inhibition of MIBG uptake due to continuously enhanced sympathetic activity. The authors concluded that this may translate into an increased risk of cardiac instability and arrhythmias.56 Finally, there are isolated examples of anti-epileptic medications worsening pre-existing cardiac pathology, for example phenytoin eliciting a previously undiagnosed Brugada syndrome72 and lamotrigine triggering a worsening of outflow tract obstruction in a patient with obstructive hypertrophic cardiomyopathy.73
Overall, there is some evidence for inter-ictal cardiac autonomic dysfunction in patients with both focal and generalised epilepsy, possibly modulated by anti-epileptic medication, in particular, carbamazepine. There are conflicting reports in the literature, however, suggesting that the relationship between inter-ictal epileptiform activity, anti-epileptic medication and autonomic function has not yet been fully characterised. This may, at least partly, be due to a lack of standardised analysis methods and heterogeneous study populations. Further work in this field is of paramount importance as the ability to stratify the risk of SUDEP in individual patients on the basis of inter-ictal autonomic parameters would have valuable management and prognostic implications.
Summary
In conclusion, it is clear that the interaction between epilepsy and the brain is complex and dynamic. Patients with epilepsy may be predisposed to the acute epileptogenic disturbance of autonomic function and subsequent cardiac arrhythmias due to the effects of recurrent seizures on cardiac microstructure, a genetically determined propensity, and/or the effects of epilepsy and anti-epileptic medication on baseline autonomic function. However, the extent, nature and temporal progression of these effects remains unclear. Although most cardiac sequelae of seizures are benign, transient and self-limiting, the heart may play a crucial role in the aetiological basis of SUDEP.
Future work should involve prospectively collected multi-centre cohort or case–control studies with standardised case ascertainment, established clinical and pathological definitions and a large number of systemic variables to further investigate the causality of SUDEP rather than incidence. Inter-ictal and ictal electrophysiological, cardiorespiratory and metabolic variables should be evaluated in a large population of patients, including in specific syndromes, to further establish the pathophysiological mechanisms of SUDEP. A key aim is to stratify the risk of SUDEP for an individual patient and, ideally, identify potential therapeutic targets. This would be augmented by general strategies for the prevention of SUDEP, including, for example, improved control of generalised tonic-clonic seizures, seizure detection and supervision.
Conflict of interest
None declared.
Editors’ note
A case report on ictal bradycardia and asystole associated with intractable epilepsy by Chaila et al. can be found on pages 245–8 of this issue.
Key Messages
- Acute neurological events, including epileptic seizures, may result in abnormalities of cardiac rhythm
- Chronic epilepsy and treatment with anti-epileptic drugs may predispose patients to the adverse autonomic effects of seizures
- Patients with epilepsy have an increased mortality rate compared with the general population, a significant proportion of which is due to sudden unexpected death in epilepsy (SUDEP)
- Proposed pathophysiological mechanisms for SUDEP include cardiac arrhythmias, apnoea and cerebral electrical shutdown
- The identification of patients at risk of ictal cardiac bradyarrhythmias, and possibly SUDEP, is an important goal as pacemaker insertion may be preventative
References
1. Goldstein DS. The electrocardiogram in stroke: relationship to pathophysiological type and comparison with prior tracings. Stroke 1979;10:253–9.
2. Rugg-Gunn FJ, Simister RJ, Squirrell M et al. Cardiac arrhythmias in focal epilepsy: a prospective long-term study. Lancet 2004;364:2212–19.
3. Blumhardt LD, Smith PE, Owen L. Electrocardiographic accompaniments of temporal lobe epileptic seizures. Lancet 1986;1:1051–6.
4. Chadwick D, Smith D. The misdiagnosis of epilepsy. BMJ 2002;324:495–6.
5. Smith D, Defalla BA, Chadwick DW. The misdiagnosis of epilepsy and the management of refractory epilepsy in a specialist clinic. QJM 1999;92:15–23.
6. Zaidi A, Clough P, Cooper P et al. Misdiagnosis of epilepsy: many seizure-like attacks have a cardiovascular cause. J Am Coll Cardiol 2000;36:181–4.
7. Samuels MA. Neurogenic heart disease: a unifying hypothesis. Am J Cardiol 1987;60:15J–19J.
8. Altenmuller DM, Zehender M, Schulze-Bonhage A. High-grade atrioventricular block triggered by spontaneous and stimulation-induced epileptic activity in the left temporal lobe. Epilepsia 2004;45:1640–4.
9. Nei M, Ho RT, bou-Khalil BW et al. EEG and ECG in sudden unexplained death in epilepsy. Epilepsia 2004;45:338–45.
10. Mayer H, Benninger F, Urak L et al. EKG abnormalities in children and adolescents with symptomatic temporal lobe epilepsy. Neurology 2004;63:324–8.
11. Galimberti CA, Marchioni E, Barzizza F et al. Partial epileptic seizures of different origin variably affect cardiac rhythm. Epilepsia 1996;37:742–7.
12. Leutmezer F, Schernthaner C, Lurger S et al. Electrocardiographic changes at the onset of epileptic seizures. Epilepsia 2003;44:348–54.
13. Zijlmans M, Flanagan D, Gotman J. Heart rate changes and ECG abnormalities during epileptic seizures: prevalence and definition of an objective clinical sign. Epilepsia 2002;43:847–54.
14. Schuele SU, Bermeo AC, Alexopoulos AV et al. Video-electrographic and clinical features in patients with ictal asystole. Neurology 2007;69:434–41.
15. Britton JW, Ghearing GR, Benarroch EE et al. The ictal bradycardia syndrome: localization and lateralization. Epilepsia 2006;47:737–44.
16. Kirchner A, Pauli E, Hilz MJ et al. Sex differences and lateral asymmetry in heart rate modulation in patients with temporal lobe epilepsy. J Neurol Neurosurg Psychiatry 2002;73:73–5.
17. Oppenheimer SM, Cechetto DF. Cardiac chronotropic organization of the rat insular cortex. Brain Res 1990;533:66–72.
18. Oppenheimer SM, Gelb A, Girvin JP et al. Cardiovascular effects of human insular cortex stimulation. Neurology 1992;42:1727–32.
19. Swartz CM, Abrams R, Lane RD et al. Heart rate differences between right and left unilateral electroconvulsive therapy. J Neurol Neurosurg Psychiatry 1994;57:97–9.
20. Espinosa PS, Lee JW, Tedrow UB et al. Sudden unexpected near death in epilepsy: malignant arrhythmia from a partial seizure. Neurology 2009;72:1702–03.
21. Russell AE. Cessation of the pulse during the onset of epileptic fits. Lancet 1906;2:152–4.
22. Smith PE, Howell SJ, Owen L et al. Profiles of instant heart rate during partial seizures. Electroencephalogr Clin Neurophysiol 1989;72:207–17.
23. Tinuper P, Bisulli F, Cerullo A et al. Ictal bradycardia in partial epileptic seizures: autonomic investigation in three cases and literature review. Brain 2001;124:2361–71.
24. Rossetti AO, Dworetzky BA, Madsen JR et al. Ictal asystole with convulsive syncope mimicking secondary generalisation: a depth electrode study. J Neurol Neurosurg Psychiatry 2005;76:885–7.
25. Devinsky O, Pacia S, Tatambhotla G. Bradycardia and asystole induced by partial seizures: a case report and literature review. Neurology 1997;48:1712–14.
26. Oppenheimer SM, Saleh T, Cechetto DF. Lateral hypothalamic area neurotransmission and neuromodulation of the specific cardiac effects of insular cortex stimulation. Brain Res 1992;581:133–42.
27. Lee KH, Meador KJ, Park YD et al. Pathophysiology of altered consciousness during seizures: subtraction SPECT study. Neurology 2002;59:841–6.
28. Rocamora R, Kurthen M, Lickfett L et al. Cardiac asystole in epilepsy: clinical and neurophysiologic features. Epilepsia 2003;44:179–85.
29. Lathers CM, Schraeder PL. Autonomic dysfunction in epilepsy: characterization of autonomic cardiac neural discharge associated with pentylenetetrazol-induced epileptogenic activity. Epilepsia 1982;23:633–47.
30. Kloster R, Engelskjon T. Sudden unexpected death in epilepsy (SUDEP): a clinical perspective and a search for risk factors. J Neurol Neurosurg Psychiatry 1999;67:439–44.
31. Falconer B, Rajs J. Post-mortem findings of cardiac lesions in epileptics: a preliminary report. Forensic Sci 1976;8:63–71.
32. Natelson BH, Suarez RV, Terrence CF et al. Patients with epilepsy who die suddenly have cardiac disease. Arch Neurol 1998;55:857–60.
33. Pirolo JS, Hutchins GM, Moore GW. Myocyte vacuolization in infarct border zones is reversible. Am J Pathol 1985;121:444–50.
34. Cordero DL, Cagin NA, Natelson BH. Neurocardiology update: role of the nervous system in coronary vasomotion. Cardiovasc Res 1995;29:319–28.
35. Natelson BH, Chang Q. Sudden death. A neurocardiologic phenomenon. Neurol Clin 1993;11:293–308.
36. Kawara T, Derksen R, de Groot JR et al. Activation delay after premature stimulation in chronically diseased human myocardium relates to the architecture of interstitial fibrosis. Circulation 2001;104:3069–75.
37. Codrea TS, ager-Pedersen S, Baandrup U et al. Sudden unexpected death in epilepsy: is death by seizures a cardiac disease? Am J Forensic Med Pathol 2005;26:99–105.
38. Sekul EA, Moak JP, Schultz RJ et al. Electrocardiographic findings in Rett syndrome: an explanation for sudden death? J Pediatr 1994;125:80–2.
39. Nashef L, Hindocha N, Makoff A. Risk factors in sudden death in epilepsy (SUDEP): the quest for mechanisms. Epilepsia 2007;48:859–71.
40. Kass RS, Moss AJ. Long QT syndrome: novel insights into the mechanisms of cardiac arrhythmias. J Clin Invest 2003;112:810–15.
41. Escayg A, MacDonald BT, Meisler MH et al. Mutations of SCN1A, encoding a neuronal sodium channel, in two families with GEFS+2. Nat Genet 2000;24:343–5.
42. Nabbout R. Can SCN1A mutations account for SUDEP? – Commentary on Hindocha et al. Epilepsia 2008;49:367–8.
43. Hindocha N, Nashef L, Elmslie F et al. Two cases of sudden unexpected death in epilepsy in a GEFS+ family with an SCN1A mutation. Epilepsia 2008;49:360–5.
44. Massetani R, Strata G, Galli R et al. Alteration of cardiac function in patients with temporal lobe epilepsy: different roles of EEG-ECG monitoring and spectral analysis of RR variability. Epilepsia 1997;38:363–9.
45. Drake ME, Reider CR, Kay A. Electrocardiography in epilepsy patients without cardiac symptoms. Seizure 1993;2:63–5.
46. Petkar S, Cooper P, Fitzpatrick A. High incidence of ECG abnormalities in severe epilepsy. World Congress of Cardiology 2006, 1335. (abstract)
47. Lathers CM, Schraeder PL, Weiner FL. Synchronization of cardiac autonomic neural discharge with epileptogenic activity: the lockstep phenomenon. Electroencephalogr Clin Neurophysiol 1987;67:247–59.
48. Frysinger RC, Engel J, Harper RM. Interictal heart rate patterns in partial seizure disorders. Neurology 1993;43:2136–9.
49. Tomson T, Ericson M, Ihrman C et al. Heart rate variability in patients with epilepsy. Epilepsy Res 1998;30:77–83.
50. Toichi M, Murai T, Sengoku A et al. Interictal change in cardiac autonomic function associated with EEG abnormalities and clinical symptoms: a longitudinal study following acute deterioration in two patients with temporal lobe epilepsy. Psychiatry Clin Neurosci 1998;52:499–505.
51. Pincus SM, Cummins TR, Haddad GG. Heart rate control in normal and aborted-SIDS infants. Am J Physiol 1993;264:R638–R646.
52. Guzzetti S, Signorini MG, Cogliati C et al. Non-linear dynamics and chaotic indices in heart rate variability of normal subjects and heart-transplanted patients. Cardiovasc Res 1996;31:441–6.
53. Bigger JT Jr., Steinman RC, Rolnitzky LM et al. Power law behavior of RR-interval variability in healthy middle-aged persons, patients with recent acute myocardial infarction, and patients with heart transplants. Circulation 1996;93:2142–51.
54. Ansakorpi H, Korpelainen JT, Suominen K et al. Interictal cardiovascular autonomic responses in patients with temporal lobe epilepsy. Epilepsia 2000;41:42–7.
55. Ansakorpi H, Korpelainen JT, Huikuri HV et al. Heart rate dynamics in refractory and well controlled temporal lobe epilepsy. J Neurol Neurosurg Psychiatry 2002;72:26–30.
56. Druschky A, Hilz MJ, Hopp P et al. Interictal cardiac autonomic dysfunction in temporal lobe epilepsy demonstrated by [(123)I]metaiodobenzylguanidine-SPECT. Brain 2001;124:2372–82.
57. Evrengul H, Tanriverdi H, Dursunoglu D et al. Time and frequency domain analyses of heart rate variability in patients with epilepsy. Epilepsy Res 2005;63:131–9.
58. Lear-Kaul KC, Coughlin L, Dobersen MJ. Sudden unexpected death in epilepsy: a retrospective study. Am J Forensic Med Pathol 2005;26:11–17.
59. Leestma JE, Annegers JF, Brodie MJ et al. Sudden unexplained death in epilepsy: observations from a large clinical development program. Epilepsia 1997;38:47–55.
60. Opeskin K, Burke MP, Cordner SM et al. Comparison of antiepileptic drug levels in sudden unexpected deaths in epilepsy with deaths from other causes. Epilepsia 1999;40:1795–8.
61. Walczak T. Do antiepileptic drugs play a role in sudden unexpected death in epilepsy? Drug Saf 2003;26:673–83.
62. Walczak TS, Leppik IE, D’Amelio M et al. Incidence and risk factors in sudden unexpected death in epilepsy: a prospective cohort study. Neurology 2001;56:519–25.
63. Hitiris N, Suratman S, Kelly K et al. Sudden unexpected death in epilepsy: a search for risk factors. Epilepsy Behav 2007;10:138–41.
64. Timmings PL. Sudden unexpected death in epilepsy: is carbamazepine implicated? Seizure 1998;7:289–91.
65. Langan Y, Nashef L, Sander JW. Case-control study of SUDEP. Neurology 2005;64:1131–3.
66. Hennessy MJ, Tighe MG, Binnie CD et al. Sudden withdrawal of carbamazepine increases cardiac sympathetic activity in sleep. Neurology 2001;57:1650–4.
67. Tomson T, Kenneback G. Arrhythmia, heart rate variability and anti-epileptic drugs. Epilepsia 1997;38:S48–S51.
68. Nilsson L, Bergman U, Diwan V et al. Antiepileptic drug therapy and its management in sudden unexpected death in epilepsy: a case-control study. Epilepsia 2001;42:667–73.
69. Isojarvi JI, Ansakorpi H, Suominen K et al. Interictal cardiovascular autonomic responses in patients with epilepsy. Epilepsia 1998;39:420–6.
70. Kenneback G, Ericson M, Tomson T et al. Changes in arrhythmia profile and heart rate variability during abrupt withdrawal of antiepileptic drugs. Implications for sudden death. Seizure 1997;6:369–75.
71. Persson H, Ericson M, Tomson T. Heart rate variability in patients with untreated epilepsy. Seizure 2007;16:504–08.
72. Al AB, Adabag AS, Houghland MA et al. Brugada pattern electrocardiogram associated with supratherapeutic phenytoin levels and the risk of sudden death. Pacing Clin Electrophysiol 2007;30:713–15.
73. Stollberger C, Hoftberger R, Finsterer J. Lamotrigine-trigged obstructive hypertrophic cardiomyopathy, epilepsy and metabolic myopathy. Int J Cardiol 2009;in press.