Introduction
Triglycerides (TG) and cholesterol are water-insoluble molecules that are transported through the aqueous medium of plasma in large quantities from sites of absorption, synthesis or storage to tissues that require them for cell functions such as energy production. To enable transport to occur, these lipids are solubilised into plasma lipoproteins which have the general structure of a lipid-filled core and a surface of phospholipid and specific proteins. It is the proteins that direct the fate of the contained lipid by interacting with key lipolytic enzymes and cell membrane receptors. Figure 1 depicts the general structure of lipoproteins and their size and density. They can be categorised by density interval into chylomicrons (density <0.95 g/mL) – intestinally derived, large TG-rich particles that appear during lipid absorption; very low-density lipoproteins (VLDL, density 0.95–1.006 g/mL) that are made by the liver on a continuous basis; low-density lipoproteins (LDL, density 1.006–1.063 g/mL) – LDL is the major cholesterol-carrying lipoprotein; lipoprotein(a), a lipoprotein of unknown function that is a complex of an LDL particle and a large protein, apo(a); and high-density lipoproteins (HDL, density 1.063–1.21 g/mL), which have a role in transporting cholesterol from peripheral tissues to the liver.
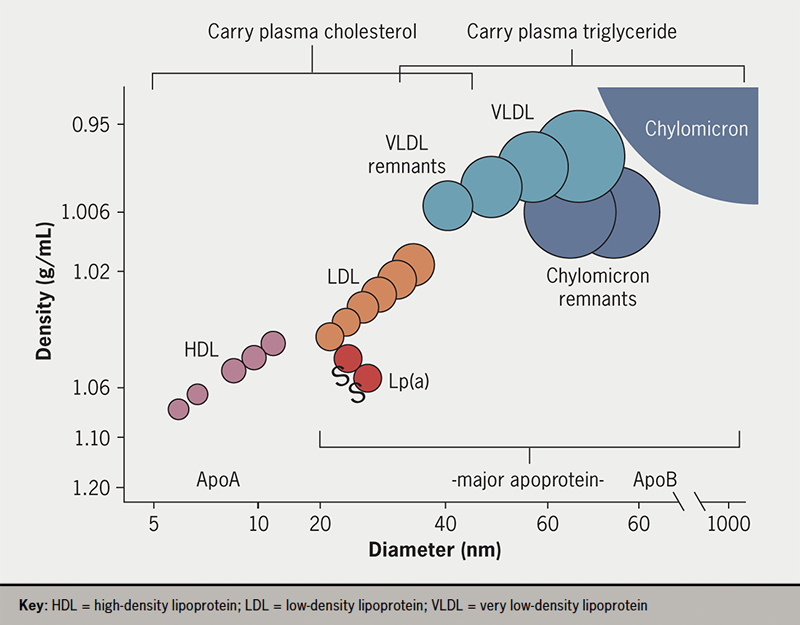
In most populations, the levels of plasma TG and cholesterol far exceed the concentrations needed to support physiological processes, which lead to pathological consequences, such as atherosclerotic cardiovascular disease (ASCVD) and pancreatitis.1,2 Additionally, LDL is recognised as a causal risk factor for ASCVD;3,4 there is a strong, positive relationship between its plasma level and ASCVD risk that extends across the range seen in the population. Intervention trials have established that LDL-lowering reduces risk and the benefits of intensive reductions in this lipoprotein are now clear.4,5 Plasma TG, carried within chylomicrons and VLDL (collectively termed triglyceride-rich lipoproteins, TRL), has long been regarded as an important risk factor for the development of atherosclerosis; however, it is only recently that evidence has emerged supporting the causal role of TRL in ASCVD.1,2,6 Specifically, genetic variants that influence the level of plasma TG (and hence TRL) are linked with an altered risk of ASCVD events.1,6,7 Triglyceride itself, unlike cholesterol, is not an abundant constituent of atherosclerotic plaques and the current working hypothesis is that it is the TG-depleted, cholesterol-enriched ‘remnants’ of chylomicron and VLDL metabolism that contribute to atherogenesis.1,2
Definition, causes and consequences of hypertriglyceridaemia
Large epidemiological studies have reported consistently that plasma TG levels are strongly associated with ASCVD risk in populations (figure 2).1,2,6,8,9 As plasma TG concentration increases, metabolic and structural perturbations arise throughout the lipoprotein spectrum; TRL remnants are generated in increasing numbers and there are changes in LDL and HDL composition, notably the formation of small, dense LDL.1,10 In a recent consensus report from the European Atherosclerosis Society,1 definitions of the degrees of hypertriglyceridaemia were developed to reflect the nature of the relationship with clinical disorders such as ASCVD and pancreatitis (table 1). Since disadvantageous changes start to become evident at TG levels below the population average (which is ~1.5 mmol/L [~133 mg/dL]), an ‘optimal’ level was defined as <1.2 mmol/L (<106 mg/dL). In this range, production and clearance rates of TRL are entirely sufficient to maintain physiological health.
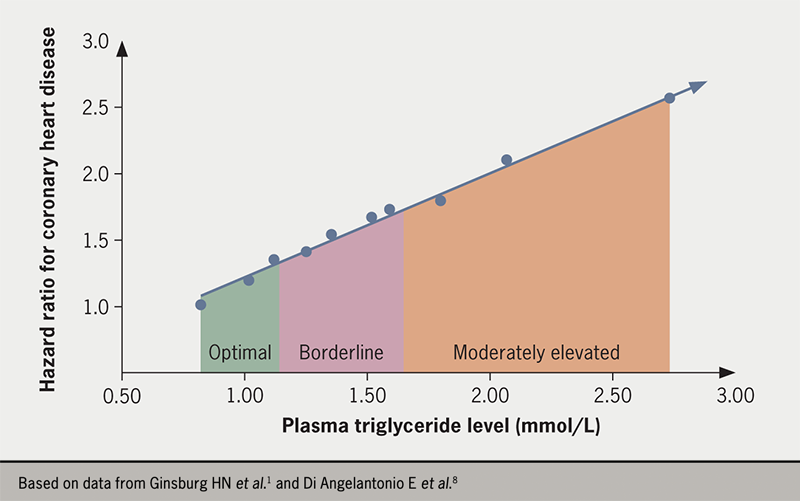
Table 1. Hypertriglyceridaemia: definition and clinical sequelae
Category | Plasma triglyceride level (mmol/L)/[mg/dL] | Primary metabolic abnormality | Clinical correlates |
---|---|---|---|
Optimal | <1.2 [<106] | ||
Borderline | 1.2–1.7 [106–150] | VLDL overproduction | Overweight ASCVD |
Moderate | 1.7–5.7 [150–504] | VLDL overproduction plus defective clearance | Obesity Fatty liver disease Type 2 diabetes ASCVD |
Severe | 5.7–10.0 [504–885] | Substantially defective clearance of VLDL and chylomicrons | Obesity Fatty liver disease Type 2 diabetes ASCVD |
Extreme | >10.0 [>885] | Combined defective lipolysis of VLDL and chylomicrons | Enlarged liver and spleen Pancreatitis |
Key: ASCVD = atherosclerotic cardiovascular disease; VLDL = very low-density lipoprotein |
‘Borderline’ hypertriglyceridaemia is classified as 1.2 to 1.7 mmol/L (106 to 150 mg/dL), while ‘moderate’ and ‘severe’ forms of the condition are defined by plasma TG concentrations in the range 1.7 to 5.7 mmol/L (150 to 504 mg/dL) and 5.7 to 10.0 mmol/L (504 to 885 mg/dL), respectively. The rationale for setting the optimal level so low is that the association with increased risk of ASCVD is continuous and graded across the range of values seen in most countries (figure 2)1,2,6,8 in analogy with the observations for LDL cholesterol (LDL-C). ‘Extreme’ hypertriglyceridaemia was defined as a TG level greater than 10.0 mmol/L (885 mg/dL), which is a risk for developing the major clinical sequela of acute pancreatitis.
Metabolic changes that give rise to hypertriglyceridaemia are a combination of TRL overproduction, slower lipolysis rates and delayed clearance of particles from the circulation.1,10 Chylomicrons are produced in a wave during absorption of dietary fat in the intestine. These particles rapidly undergo lipolysis, delivering the TGs in their core mainly to adipose tissue, while the remnants formed are cleared by the liver (figure 3). VLDL is assembled using TG released from hepatic stores or made from fatty acids taken up from the circulation. It is secreted by the liver in response to the need to have TG present in the bloodstream in the periods between meals to provide an energy source for cardiac and skeletal muscle. Being overweight or obese is associated with increased production of VLDL (table 1).11,12 Higher rates of VLDL production are also a feature of both type 2 diabetes (since insulin is a major regulator of VLDL assembly),13 and fatty liver disease (as the organ attempts to export excess fat stored in cell droplets).1,12
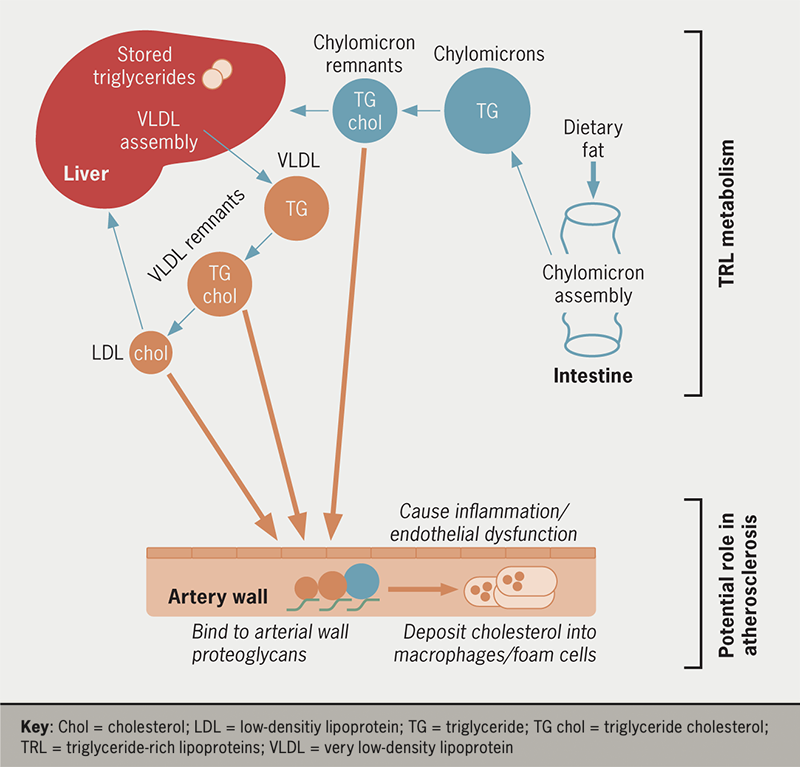
More marked degrees of hypertriglyceridaemia are caused by defects in TRL lipolysis and clearance.1,10,14,15 Impaired lipase action slows the delipidation of both chylomicrons and VLDL and increases the generation of remnant lipoproteins (figure 3). Remnants are depleted in TG in the lipid core and become enriched in cholesterol. In individuals with optimal plasma TG levels, the concentration of remnants in the circulation is low but in hypertriglyceridaemic subjects, these particles accumulate and, like LDL-C, they have the ability to be taken up and retained by the artery wall (figure 3).1,2 Remnants appear to have a number of potentially deleterious effects leading to inflammation, endothelial dysfunction, and due to their high cholesterol content per particle, can contribute to macrophage ‘foam’ cell formation (so called because of the high lipid content seen on histology) in growing atherosclerotic plaques.1
Knowledge of the metabolic aberrations that lead to elevated plasma TG levels enables an informed approach to interventions designed to lower TRL concentrations and potentially reduce the risk of ASCVD and pancreatitis. Reduction in body weight results in lower liver fat and decreased rates of VLDL production.1,11 Likewise, improved diabetic glycaemic control is associated with lower VLDL secretion rates.1,13 In an alternate approach focusing on stimulating lipolysis, a number of novel agents are in development that promote the action of lipoprotein lipase, the major enzyme responsible for TRL delipidation.7 Both intervention routes – decreased production and increased lipolysis – are likely to lower the level of atherogenic remnant particles. The major defects in lipolysis seen in extreme hypertriglyceridaemia have been addressed by agents that stimulate lipase activity.16
Approaches to lipid lowering to prevent ASCVD
Raised plasma lipid levels are strongly associated with an increased risk of ASCVD, alongside smoking and high blood pressure. Intervention trials, especially those addressing the benefits of LDL-C lowering, have underpinned the concept that the relationship is causal; lipoproteins have a direct role in the development of atherosclerotic plaques and in the precipitation of a major clinical event, such as myocardial infarction and ischaemic stroke. International guidelines have been developed that provide evidence-based recommendations regarding efficacious treatment options and therapeutic goals.4,17 These guidelines recognise the long-term nature of the disease process – atherosclerosis is a silent disease of the arterial wall that develops over decades and then manifests in the form of an acute coronary or cerebral event – and the need to promote population-based healthy lifestyle advice on diet and behaviours. Another feature of the recommendations is the adoption of a graded, risk-based approach with the aggressiveness of the intervention tailored according to the predicted ongoing risk.
Nutrition and lifestyle advice
The cornerstone intervention in both primary and secondary prevention of ASCVD is the adoption of a prudent diet and the avoidance of behaviours known to increase risk, such as smoking and sedentary lifestyle. The metabolism of cholesterol and TG is intimately linked, as shown in figure 3, and the same general advice applies to lowering both plasma lipids through dietary changes. Guideline-recommended steps for lowering plasma TG levels1,4,17 are first to reduce overall calorie intake to achieve weight loss and reduce adiposity. Second, to alter the types of fat consumed by increasing the intake of fatty fish and by substituting saturated fat (animal fat and dairy) with mono- and polyunsaturated fat sources (plant oils and nuts) and to keep the intake of trans-fatty acids to a minimum. Third, avoid low-fat, highly refined, carbohydrate-rich foods and excess consumption of alcohol. Fourth, eat larger amounts of fibre-rich foodstuffs, such as vegetables and whole grain products.
Most people with hypertriglyceridaemia fall into the overweight or obese categories and weight reduction can often produce a much greater benefit in terms of lowering plasma TG than it does in reducing LDL-C. This is in part due to the close connection between visceral and hepatic fat stores and VLDL production as outlined above.12 In people with type 2 diabetes, the initial goal is to achieve optimum glycaemic control, which in itself, can result in substantial reductions in plasma TG levels.1,4,17
Managing raised LDL-C effectively
Three decades of clinical trials have demonstrated not only that lowering LDL-C reduces the risk of major coronary and cerebral atherothrombotic events but also that the association with risk extends to very low levels of LDL.5 That is, further clinical benefit is seen when LDL-C is reduced to <1.4 mmol/L (<54 mg/dL) or even <1.0 mmol/L (<39 mg/dL) compared to previous goals of approximately 1.8 mmol/L (69.5 mg/dL). No safety issues have arisen when LDL has been profoundly lowered; potential concerns relating to haemorrhagic stroke, cognitive function and cancer were examined and no evidence was seen of treatment-emergent adverse effects of the drugs used or the low LDL level achieved. Accordingly, the latest revision of the European guidelines sets aggressive goals for LDL-C lowering of <1.4 mmol/L (<54 mg/dL) for those at highest risk, typically individuals with a history of clinical ASCVD.4
In the risk-based strategy set out in these guidelines, individuals without evidence of clinical ASCVD are first offered lifestyle and diet advice, followed by moderate-dose statin therapy for those whose LDL-C remains above the threshold for pharmacologic intervention. In secondary prevention, statin monotherapy is first-line, and international surveys show the universality of this approach.18 However, this strategy has been found to be insufficient to allow the majority of patients to meet the previous goal for LDL-C of <1.8 mmol/L (<69.5 mg/dL) and only a minority are able to reach the revised lower target level. Recognition of this fact has led to the promotion of combination LDL-lowering therapy with agents such as ezetimibe and proprotein convertase subtilisin/kexin 9 (PCSK9) inhibitors. The latter class of drugs are particularly effective in reducing LDL and with their use, most people can achieve an LDL-C of <1.4 mmol/L (<54 mg/dL), if required.4,17,19 A strategy of early use/first-line combination therapy19 recognises that treatment with statins alone, even at maximally tolerated doses, does not achieve the full benefits of lipid lowering. Where the initial LDL-C level is high, it will predictably remain above the goal on monotherapy and the early use of combined drug regimens (as in hypertension treatment) delivers the optimum benefit quickly and efficiently.
Beyond LDL – addressing residual risk on statin therapy
Clinical trial evidence shows that in secondary prevention, patients on statin monotherapy with a high residual risk can usefully be divided into (i) patients in whom LDL-C is persistently high (who require combination LDL-lowering therapy as set out above), (ii) patients with elevated plasma TG levels that contribute to ongoing risk, (iii) patients in whom lipoprotein(a) is high and (iv) those with chronic inflammation).6 Analyses from clinical trials and ‘real-world’ surveys highlight the part that TRL and their remnants play in the high residual risk in individuals on statin monotherapy.1,20 In a Canadian registry study, approximately 25% of subjects on statins had high ASCVD risk associated with elevated plasma TG levels.20 Similarly, a Danish study reported an analysis of lipid-associated predictors of ASCVD risk in participants on statins.21 Those with above-the-median non-HDL cholesterol but below-the-median LDL-C – i.e., elevated TRL/remnant cholesterol – had a high continuing risk of ASCVD.
An active question in ASCVD prevention is how best to reduce the residual risk attributable to elevated plasma TG levels (and TRL) in patients on optimised statin therapy. Clinical trials have given mixed signals. The Reduction of Cardiovascular Events With Icosapent Ethyl–Intervention Trial (REDUCE-IT) revealed that administration of high-dose purified eicosapentaenoic acid was associated with a strong positive effect, reducing the risk of ASCVD by about 25% in statin-treated participants with established, or at high risk for, ASCVD who had plasma TG levels in the range of 1.5 to 5.0 mmol/L (i.e., moderately elevated levels [133 to 442 mg/dL]).22 Other recent trials in cohorts with similar risk and lipid profiles using an eicosapentaenoic/docosahaexanoic acid mix or a fibrate type-drug gave negative results for reasons that are presently unclear.23
Summary
Raised plasma TG levels are a biomarker of the presence of an increased concentration of TRL – chylomicrons, VLDL and their remnants – in the circulation and are associated with an elevated risk of ASCVD. The relationship is continuous and graded across the range of values seen in populations and accordingly, only low levels of plasma TG (<1.2 mmol/L [<106 mg/dL]) are considered optimal. Genetic studies indicate that the relationship of TRL with disease is causal and that lowering levels of plasma TG is likely to be a useful strategy for ASCVD risk reduction, especially for individuals on statins who have a high residual risk attributable to elevated TG levels.
Key messages
- Individuals with moderately to severely elevated plasma triglyceride (TG) levels (1.7 to 10.0 mmol/L [150 to 885 mg/dL]) are at a high risk of developing atherosclerotic cardiovascular disease
- An elevated plasma TG level is a biomarker of high levels of triglyceride-rich lipoproteins (TRLs) in the circulation; regulating these is a target for intervention, especially in patients with high residual risk on statin therapy
- Diet and lifestyle interventions are the first steps required to reduce plasma TG levels; however, when this is insufficiently effective, pharmacotherapy is recommended in international guidelines to address the risk associated with raised plasma TG levels
Conflicts of interest
CP has received grants/honoraria from Amarin, Amgen, Daiichi-Sankyo, Dalcor, MSD, Novartis, Pfizer, and Response Therapeutics.
Chris J Packard
Honorary Senior Research Fellow and Professor of Vascular Biochemistry
Institute of Cardiovascular and Medical Sciences, Glasgow University, 126 University Place, Glasgow, G12 8TA
([email protected])
Articles in this supplement
Introduction
The evidence for fish oils and eicosapentaenoic acid in managing hypertriglyceridaemia
REDUCE-IT: findings and implications for practice
Icosapent ethyl use in clinical practice: current and future directions
References
1. Ginsberg HN, Packard CJ, Chapman MJ, et al. Triglyceride-rich lipoproteins and their remnants: metabolic insights, role in atherosclerotic cardiovascular disease, and emerging therapeutic strategies – a consensus statement from the European Atherosclerosis Society. Eur Heart J 2021;42:4791–806. https://doi.org/10.1093/eurheartj/ehab551
2. Nordestgaard BG, Varbo A. Triglycerides and cardiovascular disease. Lancet 2014;384:626–35. https://doi.org/10.1016/S0140-6736(14)61177-6
3. Borén J, Chapman MJ, Krauss RM, et al. Low-density lipoproteins cause atherosclerotic cardiovascular disease: pathophysiological, genetic and therapeutic insights: a consensus statement from the European Atherosclerosis Society Consensus Panel. Eur Heart J 2020;41:2313–30. https://doi.org/10.1093/eurheartj/ehz962
4. Mach F, Baigent C, Catapano AL, et al. ESC Scientific Document Group. 2019 ESC/EAS Guidelines for the management of dyslipidaemias: lipid modification to reduce cardiovascular risk. Eur Heart J 2020;41:111–88. https://doi.org/10.1093/eurheartj/ehz455
5. Packard C, Chapman MJ, Sibartie M, Laufs U, Masana L. Intensive low-density lipoprotein cholesterol lowering in cardiovascular disease prevention: opportunities and challenges. Heart 2021;107:1369–75. https://doi.org/10.1136/heartjnl-2020-318760
6. Libby P. The changing landscape of atherosclerosis. Nature 2021;592:524–33. https://doi.org/10.1038/s41586-021-03392-8
7. Tokgözoğlu L, Libby P. The dawn of a new era of targeted lipid-lowering therapies. Eur Heart J 2022;43:3198–208. https://doi.org/10.1093/eurheartj/ehab841
8. Di Angelantonio E, Sarwar N, Perry P, et al.; The Emerging Risk Factors Collaboration. Major lipids, apolipoproteins, and risk of vascular disease. JAMA 2009;302:1993–2000. https://doi.org/10.1001/jama.2009.1619
9. Sarwar N, Danesh J, Eiriksdottir G, et al. Triglycerides and the risk of coronary heart disease: 10,158 incident cases among 262,525 participants in 29 Western prospective studies. Circulation 2007;115:450–8. https://doi.org/10.1161/CIRCULATIONAHA.106.637793
10. Borén J, Taskinen MR, Björnson E, Packard CJ. Metabolism of triglyceride-rich lipoproteins in health and dyslipidaemia. Nat Rev Cardiol 2022;19:577–92. https://doi.org/10.1038/s41569-022-00676-y
11. Watts GF, Chan DC, Barrett PH, Susekov AV, Hua J, Song S. Fat compartments and apolipoprotein B-100 kinetics in overweight–obese men. Obes Res 2003;11:152–9. https://doi.org/10.1038/oby.2003.24
12. Borén J Watts GF, Adiels M, et al. Kinetic and related determinants of plasma triglyceride concentration in abdominal obesity: multicenter tracer kinetic study. Arterioscler Thromb Vasc Biol 2015;35:2218–24. https://doi.org/10.1161/ATVBAHA.115.305614
13. Taskinen M-R, Borén J. New insights into the pathophysiology of dyslipidemia in type 2 diabetes. Atherosclerosis 2015;239:483–95. https://doi.org/10.1016/j.atherosclerosis.2015.01.039
14. Taskinen M-R, Adiels M, Westerbacka J, et al. Dual metabolic defects are required to produce hypertriglyceridemia in obese subjects. Arterioscler Thromb Vasc Biol 2011;31:2144–50. https://doi.org/10.1161/ATVBAHA.111.224808
15. Baass A, Paquette M, Bernard S, Hegele RA. Familial chylomicronemia syndrome: an under-recognized cause of severe hypertriglyceridaemia. J Intern Med 2020;287:340–8. https://doi.org/10.1111/joim.13016
16. Shamsudeen I, Hegele RA. Safety and efficacy of therapies for chylomicronemia. Expert Rev Clin Pharmacol 2022;15:395–405. https://doi.org/10.1080/17512433.2022.2094768
17. Grundy SM, Stone NJ, Bailey AL, et al. 2018 AHA/ACC/AACVPR/AAPA/ABC/ACPM/ADA/AGS/APhA/ASPC/NLA/PCNA Guideline on the management of blood cholesterol: a report of the American College of Cardiology/American Heart Association Task Force on Clinical Practice Guidelines. Circulation 2019;139:e1082–143. https://doi.org/10.1161/CIR.0000000000000625
18. Ray KK, Molemans B, Schoonen WM, et al. EU-wide cross-sectional observational study of lipid-modifying therapy use in secondary and primary care: the DA VINCI study. Eur J Prev Cardiol 2021;28:1279–89. https://doi.org/10.1093/eurjpc/zwaa047
19. Ray KK, Reeskamp LF, Laufs U, et al. Combination lipid-lowering therapy as first-line strategy in very high-risk patients. Eur Heart J 2022;43:830–3. https://doi.org/10.1093/eurheartj/ehab718
20. Lawler PR, Kotrri G, Koh M, et al. Real-world risk of cardiovascular outcomes associated with hypertriglyceridaemia among individuals with atherosclerotic cardiovascular disease and potential eligibility for emerging therapies. Eur Heart J 2020;41:86–94. https://doi.org/10.1093/eurheartj/ehz767
21. Johannesen CDL, Mortensen MB, Langsted A, Nordestgaard BG. Apolipoprotein B and non-HDL cholesterol better reflect residual risk than LDL cholesterol in statin-treated patients. J Am Coll Cardiol 2021;77:1439–50. https://doi.org/10.1016/j.jacc.2021.01.027
22. Gaba P, Bhatt DL, Mason RP, Miller M, Verma S, Steg PG, Boden WE; REDUCE-IT Investigators. Benefits of icosapent ethyl for enhancing residual cardiovascular risk reduction: A review of key findings from REDUCE-IT. J Clin Lipidol 2022;16:389–402. https://doi.org/10.1016/j.jacl.2022.05.067
23. Virani SS. The fibrates story – a tepid end to a PROMINENT drug. N Engl J Med 2022;387:1991–2. https://doi.org/10.1056/NEJMe2213208