The term pharmaceutical salt is used to refer to an ionisable drug that has been combined with a counter-ion to form a neutral complex. Converting a drug into a salt through this process can increase its chemical stability, render the complex easier to administer and allow manipulation of the agent’s pharmacokinetic profile. Salt selection is now a common standard operation performed with small ionisable molecules during drug development, and in many cases the drug salts display preferential properties as compared with the parent molecule. As a consequence, there has been a rapid increase in the number of drugs produced in salt form, so that today almost half of the clinically used drugs are salts. This, combined with the increase in generic drug production, means that many drugs are now produced in more than one salt form. In almost all cases where multiple drug salts of the same agent exist, they have been marketed as therapeutically equivalent and clinicians often treat the different salt forms identically. However, in many cases this may not be justified. This review describes why many pharmaceutical salts are, in fact, not chemically equivalent, and discusses whether such chemical differences may translate into differences in therapeutic effectiveness. It will also explore, with examples, what the clinical cardiologist should consider when prescribing such agents for their patients.
Pharmaceutical salts – a clinical perspective
Economic considerations, coupled to medicine regulatory guidelines and consumer demand, have resulted in drug development being split into two main streams. The first can be considered the main business of large pharmaceutical companies, which is to provide highly novel therapies (the ‘blockbuster drugs’). These novel agents are risky to develop, but once successfully developed will more than pay for the mass of clinical testing such an agent would require, as well as for the many agents that fail to reach the marketplace. They must undergo detailed, and often highly expensive, clinical trials before their use can be licensed by the regulatory authorities.
In contrast, the second drug development stream is driven by smaller pharmaceutical companies, whose business is to re-formulate old drugs to make them safer and/or easier to use, and generic drug manufacturers that generate low-cost duplicate medicines once a drug loses patent protection. Such companies generally aim to make their therapies ‘clinically equivalent’ to the current marketed products, and this significantly reduces the further evaluation that is required prior to licensing and marketing. Clinical data required for such products do not generally need to be as detailed as for the reference products; usually, bioequivalence between the new agent and the marketed product is all that is required, as will be discussed in more detail later. When prescribing an agent from this second stream of development, clinicians may have much less information at their disposal, with rigorous efficacy and safety data only available for the original product.
When medicines have identical drug names but are simply being presented to the patient in a different form or in different packaging, this generally causes only minor problems at the bedside, usually arising from the patient believing they are getting a cheap equivalent. However, when the drug name and dose changes, this provides confusion for both the patient and prescriber. This situation is occurring more frequently now that pharmaceutical companies are increasingly using different salt forms of a drug when generating new, ‘equivalent’ medicines. Simply stating that the new and old medicines are judged to be equivalent by the regulatory authorities’ guidelines is not acceptable to many patients, and indeed may not be true in all circumstances. Here, we attempt to provide the clinician with an overview of the complex area of pharmaceutical salts and the possible therapeutic implications of using different salt preparations of given drugs, with a particular emphasis on drugs employed in cardiology.
What is a pharmaceutical salt?
A salt is the product generated upon the neutralisation of an acid or base. Pharmaceutical salts are important in the process of drug development, as converting an acidic or basic drug into a salt via a simple neutralisation reaction has the ability to change the physicochemical properties of a drug. Using different chemical species to neutralise the parent drug can produce a diverse series of compounds, and this process is traditionally used to improve drug solubility and drug dissolution rates.1 The type of salt is usually categorised by the bond between the neutralisation agent and the parent drug. Salts can be broadly classified into two groups, covalent and ionic.
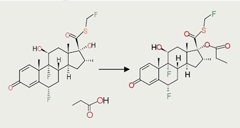
Covalent-bonded salts are formed when the parent drug and the neutralisation agent are held together by the sharing of electrons. The propionates are examples of covalently bound salts that are used to increase the lipophilicity of corticosteroids, in order to aid their retention in the airways when given by inhalation (figure 1).2-4 The properties of fluticasone propionate are retained in both the solid and liquid state, as the propionate salt is chemically bonded to the fluticasone base, thereby guaranteeing robust pharmacological and clinical activity in vivo.
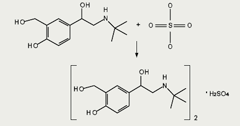
In contrast, ionic salts, which are formed by a chemical attraction of the oppositely charged parent drug and neutralising compound, do not retain their chemical identity in solution (figure 2). Ionic bonds can be readily broken and reformed in polar solutions, and this equilibrium is heavily influenced by the environmental conditions in the fluid in which the drug is dissolved.5 The well-characterised properties of ionic salts in the solid state do not necessarily reflect their properties in solution, where bonds can be formed and re-formed in the presence of other ions.
Pharmaceutically active species that contain both multiple acidic and basic functional groups in the same molecule complicate salt formation even further. Zwitterionic actives (i.e. drugs that have at least one acidic and one basic ionisable functional group, e.g. salbutamol sulphate) can potentially form both acidic and basic salts depending upon their environment, and again the species most commonly exist in a dynamic equilibrium when dissolved in simple aqueous buffers.6
An understanding of the type of drug salt being administered to a patient is critical to predicting its behaviour, but this information is not obvious to most prescribers from the salt name. It is, therefore, important that the prescriber understands the essential principle that salts formed by covalent attachment of a counter-ion and those formed by ionic association of the counter-ion present two very different situations when administered to a patient. The drug name can provide a clue as to the type of salt, as small charged molecules such as sulphate, hydrochloride, nitrate, etc. are all only ever used to form ionic drug salts, but as the counter-ions get bigger this is less intuitive and the basic chemical information must be sought.
Once the type of salt has been identified, the consequences of the salt type need to be considered. As the covalent salts are formed from the generation of a new chemical bond, they are robust molecules and, when compared against either the parent chemical entity (the original molecule used to make the salt) or an alternative salt form of the same molecule, they display distinct chemical properties, and, hence, must be considered as distinct chemical entities. Covalent salts should, therefore, generally be treated as individual agents, and very few such salts of the same parent molecule are bioequivalent. It is the ionic drug salts that can exhibit variability in absorption, pharmacokinetics, drug action and excretion. Clinicians, therefore, need to understand these salts, in particular, to appreciate the possible therapeutic consequences of prescribing different salt formulations, and, hence, these agents form the focus of this review.
Clinical equivalence (or otherwise) of salts
The basis for assessing if two agents are likely to have an equivalent effect when treating a pathological condition is the data generated from pharmacokinetic studies. This assumes that equivalent drug presence in the blood equates to equivalent drug action, and is the paradigm of most bioequivalence testing. The bioequivalence of two agents, which can be defined as the absence of a significant difference in the rate and extent to which the active ingredient or active moiety becomes available at the site of action when administered at the same molar dose under similar conditions,7 is dependent on the assessment of drug bioavailability, i.e. the rate and extent to which the active ingredient in a drug product becomes available at the site of action. It is what governs therapeutic response to a drug and is reliant on a number of factors, not least of which is the nature of the drug and the form in which it is presented. Bioequivalence studies are performed in order to ensure pharmaceutical quality, which usually also implies establishing therapeutic performance, of a drug product. Such studies are usually conducted when the patent life of a proprietary or branded drug expires and generic forms of the drug become available for substitution. Thus, a drug product is considered a pharmaceutical equivalent if it contains the same active ingredient(s), has the same dosage form and route of administration, and is identical in strength or concentration. Equivalent products contain the same amount of ingredient in the same dosage form but may differ in characteristics, such as shape, release mechanisms, and packaging.
Generating a chemically identical salt of a drug generically once its patent has expired will normally entail bioequivalence testing prior to marketing approval being sought. The release of a generic alternative to a blockbuster drug is now common, the use of such a product can be substantiated and it provides few problems among clinicians and patients if they are counselled about the switch in packaging. However, upon expiry of their patent protection, several drugs are developed in an alternative salt form. These medicines look like generic products in terms of packaging, and they arrive in the market place at the same time as generics would. However, technically they are no longer defined as pharmaceutical equivalents, but rather as pharmaceutical alternatives. One might assume that such alternatives would require a full clinical evaluation package, and, hence, might be much more costly to develop than a generic, however, this is usually not the case. Such alternative salt forms of marketed drugs may allow dramatic improvements in their synthesis or the processability of the formulations, thus leading to considerable reductions in manufacturing costs.
Both Food and Drug Administration (FDA) and European Medicines Agency (EMEA) guidance7,8 state that bioequivalence should be established between two drug products which are pharmaceutical alternatives. Bioequivalence data that can be used to support the marketing registration of alternative salt forms of a therapeutic can be demonstrated through a range of in vivo or in vitro test methods, comparative clinical trials, or pharmacodynamic studies. For example, bioequivalence can be established on the basis of the maximum serum concentration of the drug (Cmax), the time until maximum concentration is reached (tmax), or the area under the curve based on serum concentration as a function of time (AUC). Bioequivalence testing has been comprehensively reviewed by Meredith.9 Verbeek et al.10 elegantly showed that establishing bioequivalence between drug products using the current guidelines is not sufficient in many cases to demonstrate therapeutic equivalence, and suggested that further clinical data should be gathered. Nonetheless, since such data are not normally required by the regulatory authorities, this provides medicine manufacturers with the option of producing a reduced clinical evaluation package for such therapeutic alternatives. This is a worrying situation when extended to new salt forms of a therapeutic agent, as these compounds can display radically different chemical properties to their proposed equivalent. From a clinician’s point of view, it is important to consider that the definition of bioequivalence when applied to varying salt forms of a drug is very different to that when applied to a generic drug, and this may have important consequences when selecting medicines for clinical use.
When are salts likely not to be chemically equivalent?
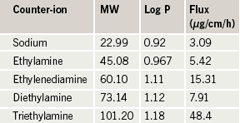
As the properties of an ionic drug salt can be modified simply by switching the counter-ion used to produce the salt, this characteristic has been utilised by pharmaceutical companies to generate different formulations that can be used to treat different indications and alter the drug’s pharmacokinetics when using the same active ingredient.6,11 Altering the chemical properties of a pharmaceutical active such as log P (partition coefficient), pKa (dissociation constant) and melting point by salt formation will influence the behaviour of the parent drug in the body through alterations in drug solubility, dissolution and stability. For example, the type of counter-ion used to prepare an ionic drug salt will affect the log P value of the administered active. Log P, a measure of the relative affinity of a chemical between a hydrophobic and hydrophilic vehicle, dictates the drug’s absorption and distribution within the body.12 Ibuprofen can be used to illustrate the impact that changing the salt counter-ion can have on log P and membrane absorption (table 1).13
An increase in log P for ibuprofen salts correlates well with an increase in the molecular weight of the counter-ion employed to form the drug salt, and this chemical property has been shown to alter the rate of absorption (flux) across biological membranes.13 The reasons behind the selection of a certain drug counter-ion may not always be obvious to the clinician using the agent. In some circumstances, it is necessary to prepare specific counter-ions to resolve specific problems with the parent drug. For example, the analgesic propoxyphene was initially formulated as a hydrochloride salt and co-administered with aspirin. However, when propoxyphene hydrochloride came into close contact with aspirin, the compound became unstable. Propoxyphene was reformulated as the napsylate salt, which showed no indication of instability, and a second formulation was generated.14 As another example, perindopril, an angiotensin-converting enzyme (ACE) inhibitor widely used in cardiovascular therapeutics, is available as a salt of tert-butylamine (erbumine), which is freely soluble in water and in ethanol (96%), and has a shelf-life of approximately two years. However, it is known that perindopril requires special packaging in countries with high temperature and relative humidity, and Telejko15 reported that the arginine salt, in comparison with the erbumine salt, was more stable and led to a 50% increase in shelf-life, an important step for distribution of this drug in the developing world. The safety profile of the parent drug can also be altered by switching an ionic salt counter-ion; for example the acute oral toxicity of propoxyphene was halved when prepared as the napsylate salt rather than the hydrochloride salt.14
An appreciation of how the nature of a counter-ion can influence the properties of a drug allows clinicians to assess how an agent may behave in a particular set of circumstances. For example, it is unlikely that a drug that is highly soluble in water and rapidly absorbed and excreted will be affected by the switching of one small molecular weight drug salt counter-ion to another. However, the use of large counter-ions (for example, xinafoate) will alter the permeation of the therapeutic agent across a biological membrane, as well as the drug’s solubility and dissolution, and, thus, could affect its duration of action, onset of action and clearance rate. Several examples specific to cardiology that involve these principles will be discussed in the next section.
The clinical implications of using different pharmaceutical salts: relevance to cardiological practice
For the clinician working with cardiovascular patients, the greatest concern with salt switching relates to the safety and efficacy of agents with a narrow therapeutic index, e.g. sotalol hydrochloride and warfarin sodium. However, a review of the literature reveals that no comparative clinical investigations into drug salt switching for such agents have been performed. Nonetheless, a number of safety concerns have arisen. For example, Olovson et al.16 showed that the aqueous solubility and dissolution rate of different alprenolol salt forms when administered via the oral route can induce variation in the degree of gastrointestinal irritation and/or ulceration. They investigated the ulcerogenic effects of five different salts in a porcine oesophageal model. The salts with the highest water solubility (hydrochloride and fumarate) gave the most serious oesophageal lesions, whereas the low-solubility salts (benzoate, maleate and sebacate) produced no irritant effects. Positive benefits of drug salt switching have also been reported. Telejko15 reported, using an open-label, randomised, two-period, cross-over, pharmacokinetic study in healthy male volunteers, that although no pharmacokinetic differences (Cmax, tmax, AUC and half-life [t1/2]) were observed between the arginine and the erbumine salts of perindopril, there were half as many reports of adverse events when using the arginine salt. It should also be noted that the change in perindopril salt in these studies also resulted in a 25% change in dose of the parent drug as a function of the change in molecular weight, another important practical consideration for prescribers.
In terms of cardiovascular drug efficacy, various studies have assessed clinical equivalence using parameters such as heart rate or blood pressure. Kim et al.17 compared amlodipine salt forms in a multi-centre, double-blind, parallel-group, randomised, controlled trial, and found that the amlodipine besylate (Norvasc) and camyslate salts, were equivalent in terms of tolerability. Lee et al.18 also studied two amlodipine salt formulations, adipate and besylate, in patients with mild-to-moderate hypertension. In a randomised, double-blind, parallel-group study, they found that the adipate salt had comparable tolerability and efficacy to the marketed besylate salt, with the primary efficacy measure being mean change from baseline in trough diastolic blood pressure after eight weeks of treatment. Although the conclusions from the pharmacokinetic data in this study indicated salt bioequivalence, a report filed to the FDA by Pfizer Inc. against the manufacturer of the maleate salt stated that the maleate was not considered therapeutically equivalent because the instability of the maleate salt resulted in the formation of a degradation product with some demonstrable clinical activity.19
Prasugrel provides an important example of how the salt formulation can lead to therapeutic differences, especially when other drugs are co-administered. During the development of prasugrel, it was noted that the bioavailability of the hydrochloride salt was greater at higher gastric pH than that of the free base.20 Since proton-pump inhibitors may often be co-prescribed with prasugrel, this observation becomes quite relevant, both pharmaceutically and clinically. It was also noted that, during manufacture, there was an in-process conversion from the salt to the base through an acid–base reaction. This led to an examination of the effect of this salt-to-base conversion on bioavailability.
Two bioequivalence studies were conducted to compare the bioavailability of lots with low (5%), intermediate (58%), and high (70%) degrees of conversion to base, with and without co-administration of lansoprazole. It was demonstrated that, when prasugrel 60 mg was administered on a background of lansoprazole, lots with low, intermediate and high salt conversion were bioequivalent for the active metabolite with respect to AUC, but were not bioequivalent with respect to Cmax: the mean difference in Cmax between low and high conversion lots was 29%, and there was a 20% mean difference in Cmax between medium and high conversion lots. In terms of pharmacodynamics, the salt-to-base conversion manifested as a delay in achieving maximal inhibition of platelet aggregation. This was significant only for high salt-to-base conversion, and only in the presence of the proton-pump inhibitor; this is likely to be important clinically, since it is considered crucial to achieve rapid platelet inhibition following acute myocardial infarction.
Case study – clopidogrel
Clopidogrel is a thienopyridine inhibitor of adenosine diphosphate-induced platelet activation, and is used (in addition to aspirin, or as an alternative in patients intolerant of aspirin) in the prevention of arterial thrombosis in patients considered at high risk. It is marketed worldwide as the bisulphate (hydrogen sulphate) salt form, of which there are approximately 20 different brands marketed worldwide. The free base of clopidogrel is unstable due to a labile proton leading to hydrolysis and degradation, therefore, it has been formulated as a salt. Clopidogrel is a prodrug that requires conversion in the liver by several CYP proteins, including CYP3A4 and CYP2C19, to its active metabolite (carboxylic acid derivative, SR 26334).21 In patients taking clopidogrel, there is a large inter-individual variability in platelet response, and in some cases poor responses have been reported, with genetic polymorphisms between individuals in clopidogrel metabolism being considered important.22-25 It has been shown that patients carrying two CYP2C19 loss-of-function alleles exhibited a worse clinical outcome (rates of cardiovascular events) when treated with clopidogrel after acute myocardial infarction (due to lower plasma concentrations of the active metabolite).24,25 In the UK, clopidogrel bisulphate is marketed as Plavix (Sanofi Aventis); however, in 2008 a German generics maker, Hexal, launched its own version of clopidogrel after a German court backed its launch in spite of opposition from Sanofi Aventis. This version of clopidogrel is a besylate salt, and it was reported by Hexal that this salt was bioequivalent to the bisulphate. Indeed, a study by Kim et al.26 investigated the relative bioavailability, pharmacokinetics and pharmacodynamics of the bisulphate and besylate salts, and found the besylate salt to be equivalent to the bisulphate in all these parameters. However, because the bisulphate salt is not completely devoid of the degradation problems associated with the parent drug, several alternative salt forms of clopidogrel are still attracting attention. One study by Ki et al.27 examined the efficacy and safety of clopidogrel resinate compared with the bisulphate salt. They performed a single dose clinical study in beagle dogs and measured pharmacokinetic (Cmax, tmax and AUC) as well as pharmacodynamic changes (platelet aggregation and bleeding time). Under their test conditions, they found bioequivalence of the resinate and bisulphate salts.
Despite the reports of bioequivalence between different clopidogrel salts, it is still unclear if there is true therapeutic equivalence. Indeed, there is some evidence that supports variable absorption as a prominent factor in the inter-individual variability seen in response to clopidogrel.28 These issues become of particular importance in light of the fact that the EMEA29 has adopted positive opinions for 13 generic forms of three different salts of clopidogrel: hydrogen sulphate (two), besylate (four) and hydrochloride (seven). Reduced antiplatelet activity of clopidogrel has also been reported in the presence of proton-pump inhibitors, and the favoured explanation relates to the action of many proton-pump inhibitors as inhibitors of CYP2C19.30 However, other reports have disputed such an effect,31 not least because such an effect is also seen with proton-pump inhibitors that appear to have no effect on CYP2C19 or indeed other CYP isoforms, e.g. pantoprazole.
It is conceivable that the inter-individual differences observed in clopidogrel activity and the interactions observed with proton-pump inhibitors relate to changes in gastric acid production, which will have an important impact on the ionisation status of clopidogrel salts (thus affecting the drug’s absorption), and this impact would be predicted to vary depending on the salt used. For a drug to be absorbed, in the absence of an active uptake mechanism, it must be sufficiently lipophilic to cross a biological membrane. For an ionisable drug, it must either be present in a fluid at an appropriate pH to suppress the ionisation of the molecule (this equates to be at a pH two units above the pKa of the molecule for an acid or two pH units below the pKa for a base) or it must form an ion-pair with a counter-ion (as discussed previously). At the time of writing, clopidogrel is available in three salt forms and, if the molecule does form an ion-pair to facilitate absorption under certain physiological conditions, this may result in differences in relative permeabilities and, hence, absorption between the salts, which may become more evident by concomitant addition of a proton-pump inhibitor.
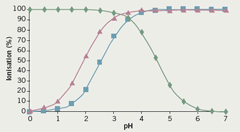
Clopidogrel, the parent drug, is a basic ionisable molecule and has a pKa value of 4.55; the bisulphate, besylate and hydrochloride counter-ions have pKa values of 1.92, 2.54 and -6.1 respectively. Figure 3 shows how the drug and counter-ion ionisation changes for the bisulphate and besylate counter-ions compare with the parent drug when solution pH is adjusted. The hydrochloride counter-ion is not shown, as its dissociation translates into the counter-ion always being ionised regardless of the pH.
From this graph, it is evident that the clopidogrel base is likely to be absorbed into the systemic circulation via the small intestine, since it will be unionised when the pH in the gastrointestinal tract reaches 6; on the other hand, absorption of an ion-pair between the drug and its salt in solution would be possible between pH 1 and 5, i.e. where both the drug and counter-ion are ionised. As the two counter-ions have a different dissociation constant, the amount of ion-pair at any given pH would be different, and this could lead to significant differences in bioavailability. It is interesting to note that one of the most significant consequences of co-administration of proton-pump inhibitors is their ability to markedly alter the pH of the stomach, from pH 1–5 over time; such an alteration would have consequences for both drug and counter-ion ionisation, which again may affect absorption of the drug in the gastrointestinal tract.
Conclusions
We have aimed to explain what pharmaceutical salts are, their role in drug development, their properties (chemical and biological), how different drug forms and salts are determined to be equivalent and the regulatory issues involved, and, more importantly, the concept that pharmaceutical equivalence (bioequivalence) does not necessarily equate to therapeutic equivalence. From a clinical perspective, an understanding of drug properties is essential in order to appreciate possible effects on therapeutic outcome (in terms of both patient safety and clinical efficacy) when changing from one drug form to another (generic substitution), and especially from one salt form to another. This is important when prescribing new versions of an existing drug; and for those drugs with narrow therapeutic indices, or with known unpredictable clinical effects or high inter-individual variability, it is essential that effective and robust monitoring is in place to ensure patient safety and to ascertain clinical response, regardless of demonstration of pharmaceutical equivalence.
Conflict of interest
None declared.
Key messages
- Many drugs are available in more than one salt form. These are not always chemically equivalent and this, in turn, may translate into differences in therapeutic effectiveness
- Such differences are especially important for drugs with narrow therapeutic indices, or with known unpredictable clinical effects or high inter-individual variability
- In some cases, a change in salt formulation of a drug can also result in a significant change in molecular weight of the compound, which in turn will necessitate a change in prescribed dose
References
- Serajuddin ATM. Salt formation to improve drug solubility. Advanced Drug Delivery Reviews 2007;59:603–16.
- Derendorf H, Meltzer EO. Molecular and clinical pharmacology of intranasal corticosteroids: Clinical and therapeutic implications. Allergy: European Journal of Allergy and Clinical Immunology 2008;63:1292–300.
- Johnson M. Development of fluticasone propionate and comparison with other inhaled corticosteroids. J Allergy Clin Immunol 1998;101(4 Pt 2):S434–S439.
- Crim C, Pierre LN, Daley-Yates PT. A review of the pharmacology and pharmacokinetics of inhaled fluticasone propionate and mometasone furoate. Clin Ther 2001;23:1339–54.
- Berge SM, Bighley LD, Monkhouse DC. Pharmaceutical salts. J Pharm Sci 1977;66:1–19.
- Stahl and Wermuth. Handbook of pharmaceutical salts: properties, selection, and use. Weinheim: Wiley-VCH, 2002.
- Food and Drug Administration. Chapter 1—Food and Drug Administration, Department of Health and Human Services. Part 320—Bioavailability and bioequivalence requirements. Available from: http://www.access.gpo.gov/nara/cfr/waisidx_00/21cfr320_00.html [accessed 20 June 2009].
- European Medicines Authority. Guideline on the investigation of bioequivalence (CPMP/EWP/QWP/1401/98 Rev. 1). Available from: http://www.emea.europa.eu/pdfs/human/qwp/140198enrev1.pdf [accessed 20 June 2009].
- Meredith P. Bioequivalence and other unresolved issues in generic drug substitution. Clin Ther 2003;25:2875–90.
- Verbeek RK, Kanfer I, Walker RB. Generic substitution: the use of medicinal products containing different salts and implications for safety and efficacy. Eur J Pharm Sci 2006;28:1–6.
- Gould PL. Salt selection for basic drugs. Int J Pharm 1986;33:201–17.
- Leo A, Hansch C, Elkins D. Partition coefficients and their uses. Chem Rev 1971;71:525–616.
- Sarveiya V, Templeton JF, Benson HAE. Ion-pairs of ibuprofen: increased membrane diffusion. J Pharm Pharmacol 2004;56:717–24.
- Davies G. Changing the salt, changing the drug. Pharm J 2001;266:322–3.
- Telejko E. Perindopril arginine: benefits of a new salt of the ACE inhibitor perindopril. Curr Med Res Opin 2007;23:953–60.
- Olovson S-G, Havu N, Regardh C-G et al. Oesophageal ulcerations and plasma levels of different alprenolol salts: potential implications for the clinic. Acta Pharmacol Toxicol 1986;58:55–60.
- Kim SH, Kim YD, Lim DS et al. Results of a phase III, 8-week, multicenter, prospective, randomized, double-blind, parallel-group clinical trial to assess the effects of amlodipine camsylate versus amlodipine besylate in Korean adults with mild to moderate hypertension. Clin Ther 2007;29:1924–36.
- Lee H-Y, Kang H-Y, Koo B-K et al. Clinic blood pressure responses to two amlodipine salt formulations, adipate and besylate, in adult Korean patients with mild to moderate hypertension: a multicentre, randomized, double-blind, parallel-group, 8-week comparison. Clin Ther 2005;27:728–39.
- Food and Drug Administration. Amlodipine citizen petition. Available from: http://www.fda.gov/ohrms/dockets/dailys/03/Sept03/090303/03p-0408-cp00001-08-Tab-G-vol3.pdf [accessed 20 June 2009].
- Center for Drug Evaluation and Research (CDER). Division of cardiovascular and renal products. Revised secondary CDTI review (2009). Prasugrel hydrochloride. Available from: http://cardiobrief.files.wordpress.com/2009/02/original-fda-prasugrel-review-document.pdf [accessed 20 June 2009].
- Clarke TA, Waskell LA. The metabolism of clopidogrel is catalyzed by human cytochrome P450 3A and is inhibited by atorvastatin. Drug Metab Dispos 2003;31:53–9.
- Angiolillo DJ, Fernandez-Ortiz A, Bernardo E et al. Contribution of gene sequence variations of the hepatic cytochrome P450 3A4 enzyme to variability in individual responsiveness to clopidogrel. Arterioscler Thromb Vasc Biol 2006;26:1895–900.
- Brandt JT, Close SL, Iturria SJ et al. Common polymorphisms of CYP2C19 and CYP2C9 affect the pharmacokinetic and pharmacodynamic response to clopidogrel but not prasugrel. J Thromb Haemost 2007;5:2429–36.
- Giusti B, Gori AM, Marcucci R et al. Cytochrome P450 2C19 loss-of-function polymorphism, but not CYP3A4 IVS10 + 12G/A and P2Y12 T744C polymorphisms, is associated with response variability to dual antiplatelet treatment in high-risk vascular patients. Pharmacogenet Genomics 2007;17:1057–64.
- Trenk D, Hochholzer W, Fromm MF et al. Cytochrome P450 2C19 681G>A polymorphism and high on-clopidogrel platelet reactivity associated with adverse 1-year clinical outcome of elective percutaneous coronary intervention with drug-eluting or bare-metal stents. J Am Coll Cardiol 2008;51:1925–34.
- Kim S, Lee H, Kwan E et al. Relative bioavailability, pharmacokinetics and pharmacodynamics of a new clopidogrel formulation in healthy male volunteers. Clin Pharmacol Therap 2008;83(suppl 1):S59.
- Ki M-H, Choi M-H, Ahn K-B et al. The efficacy and safety of clopidogrel resinate as a novel polymeric salt form of clopidogrel. Arch Pharm Res 2008;31:250–8.
- Taubert D, Kastrati A, Harlfinger S et al. Pharmacokinetics of clopidogrel after administration of a high loading dose. Thromb Haemost 2004;92:311–16.
- European Medicines Agency. Committee for medicinal products for human use June 2009 plenary meeting monthly report. Available from: http://www.emea.europa.eu/pdfs/human/press/pr/38932609en.pdf [accessed 15 July 2009].
- Gilard M, Arnaud B, Cornily J-C et al. Influence of omeprazole on the antiplatelet action of clopidogrel associated with aspirin: the randomized, double-blind OCLA (Omeprazole Clopidogrel Aspirin) study. J Am Coll Cardiol 2006;51:256–60.
- Small DS, Farid NA, Payne CD et al. Effects of the proton pump inhibitor lansoprazole on the pharmacokinetics and pharmacodynamics of prasugrel and clopidogrel. J Clin Pharmacol 2008;28:475–84.