The utility of computed tomography (CT) coronary angiography (CTCA) is underpinned by its excellent sensitivity and negative-predictive value for coronary artery disease (CAD), although it lacks specificity. Invasive coronary angiography (ICA) and invasive fractional flow reserve (FFR), are gold-standard investigations for coronary artery disease, however, they are resource intensive and associated with a small risk of serious complications. FFR-CT has been shown to have comparable performance to FFR measurements and has the potential to reduce unnecessary ICAs. The aim of this study is to briefly review FFR-CT, as an investigational modality for stable angina, and to share ‘real-world’ UK data, in consecutive patients, following the initial adoption of FFR-CT in our district general hospital in 2016.
A retrospective analysis was performed of a previously published consecutive series of 157 patients referred for CTCA by our group in a single, non-interventional, district general hospital. Our multi-disciplinary team (MDT) recorded the likely definitive outcome following CTCA, namely intervention or optimised medical management. FFR-CT analysis was performed on 24 consecutive patients where the MDT recommendation was for ICA. The CTCA + MDT findings, FFR-CT and ICA ± FFR were correlated along with the definitive outcome.
In comparing CTCA + MDT, FFR-CT and definitive outcome, in terms of whether a percutaneous coronary intervention was performed, FFR-CT was significantly correlated with definitive outcome (r=0.471, p=0.036) as opposed to CTCA + MDT (r=0.378, p=0.07). In five cases (21%, 5/24), FFR-CT could have altered the management plan by reclassification of coronary stenosis. FFR-CT of 60 coronary artery vessels (83%, 60/72) (mean FFR-CT ratio 0.82 ± 0.10) compared well with FFR performed on 18 coronary vessels (mean 0.80 ± 0.11) (r=0.758, p=0.0013).
In conclusion, FFR-CT potentially adds value to MDT outcome of CTCA, increasing the specificity and predictive accuracy of CTCA. FFR-CT may be best utilised to investigate CTCAs where there is potentially prognostically significant moderate disease or severe disease to maximise cost-effectiveness. These data could be used by other NHS trusts to best incorporate FFR-CT into their diagnostic pathways for the investigation of stable chest pain.
Introduction
Computed tomography (CT) coronary angiography (CTCA) is the National Institute for Health and Care Excellence (NICE) recommended1 first-line investigation for patients with typical or atypical chest pain who have no previous diagnosis of coronary artery disease (CAD). The clinical utility of this imaging modality is underpinned by its excellent sensitivity (99%) and negative-predictive value (97%) for CAD.2 However, CTCA lacks specificity for clinically significant CAD.3,4 CTCA overestimates occlusive plaque disease, with less than half of severe stenoses causing ischaemia.3 This has led to concerns that a CTCA approach alone, while decreasing the number of patients referred for ICA with non-obstructed coronary arteries, may increase the overall number of ICAs in comparison to other non-invasive imaging modalities.5-8 Even high-grade lesions identified on CTCA, confirmed with invasive coronary angiography (ICA), still only cause ischaemia in the minority.3,9
The current strategy for the management of CAD is guided either by the presence of significant anatomical luminal narrowing during ICA, or physiologically; by the mean pressure difference across a coronary artery stenosis. Because flow is proportional to pressure, if resistance is minimal and constant, pressure can be used as a surrogate of flow during maximal hyperaemia. There should be no pressure difference between the coronary artery and aortic root in the absence of coronary stenosis, so a reduction in pressure, distal to a stenosis, can be used as a surrogate for ischaemia and subsequently guide revascularisation.10 Initially, an FFR ratio of <0.75 was the threshold for revascularisation, as it was associated with a >99% positive predictive value for inducible myocardial ischaemia across multiple non-invasive modalities. The FFR threshold to guide revascularisation was increased to 0.80 to improve its sensitivity in detecting ischaemia, at the cost of specificity, as a small proportion of lesions with an FFR between 0.75 and 0.80 are associated with flow limitation.11 ICA, particularly in conjunction with FFR, is a resource-intensive investigation, associated with a small risk of serious complications.
The application of computational flow dynamics to CTCA enabled the calculation of coronary flow and pressure from an anatomical CTCA scan.12 HeartFlow (HeartFlow Inc., Redwood City, California) developed the first clinically used algorithm for FFR-CT and subsequently has the most established evidence-base.13-16 FFR-CT has been shown to have comparable performance to FFR measurements,13,14,17,18 and prognostically an FFR-CT of <0.8 is associated with a significantly higher risk of myocardial infarction, death and revascularisation.19-21 The FFR value is calculated from the myocardial wall volume used to derive the myocardial mass, the brachial blood pressure (assumed to be equivalent to the aortic root pressure), total coronary flow (calculated from the proportional relationship with myocardial mass) and total coronary resistance. Millions of equations are then solved using a computational method to give the pressure and velocity along the coronary vessels. Models of maximal hyperaemia are then applied.16 This gives a FFR-CT value, with pressure at the most distal part of the vessel, for each coronary artery system: right coronary artery (RCA), left anterior descending (LAD) and circumflex (Cx). Several companies have developed differing techniques to derive a non-invasive FFR measure from a CTCA including Siemens22,23 and Toshiba.24
In February 2017, NICE produced medical technological guidance on the use of HeartFlow FFR-CT with approval for use as an option for patients who were offered CTCA as part of the NICE pathway for stable chest pain. NICE concluded a per-patient saving of £214 compared with current diagnostic pathways.25 In another study, a model utilising FFR-CT for patients with a pre-test likelihood (Diamond Forrester criteria) of 10–90% predicted a 48% and 49% reduction in invasive angiography and percutaneous coronary intervention (PCI), a saving of £200 per patient and a reduction in relative adverse event rates of 4%.26 However, the cost-effectiveness of FFR-CT has been assessed in the US and, while there was a significantly lower mean and median cost from a FFR-CT guided strategy than a usual invasive strategy, there was no cost saving in comparison with non-invasive strategies,4 albeit with a differing health economy to the UK. This may indicate that FFR-CT is best utilised, in terms of cost-effectiveness, in patients who have moderate-to-severe lesions. Currently, UK hospital trusts are not required to pay the costs of FFR-CT.
We have previously highlighted the benefits of a joint cardiology/radiology multi-disciplinary team (MDT) approach to reporting CTCA.25 The aim of this study, further to a brief review of FFR-CT, was to share ‘real-world’ UK data, in consecutive patients, following the initial adoption of FFR-CT in our district general hospital in 2016.
Methods
A retrospective analysis was completed of a previously published27 consecutive series of 157 CTCAs (from 162 referrals), performed between August 2015 and February 2016. The methods of referral screening, pre-check procedure, CTCA acquisition, reporting, MDT involvement and patient demographics have been previously described.27
Pre-test investigations, CTCA protocol, and calcium score (CACS), were noted. Each CTCA was (retrospectively) categorised into 0–50% stenosis (normal or non-flow limiting, NFL), 50–70% (possible flow limiting, PFL) or >70% (critical stenosis, CS) as based on previously described definitions27 realigned to CAD-RADS (coronary artery disease – reporting and data system) criteria.28 The coronary arteries were subdivided into ‘proximal’, accounting for the first third of the vessel length from origin, and ‘other’ accounting for the final two thirds of the coronary artery or sub-branch (e.g. marginal branch or diagonal branch). The MDT, at the time, recorded its recommendation for further care, namely: optimal medical therapy (OMT) and ICA with likely PCI or OMT alone. The culprit vessel(s) were also identified and noted at MDT.
Following CTCA, 24% (n=37) of patients were referred for ICA based on prior described pathways.27 Of these, 24 patients had an FFR-CT (HeartFlow) performed consecutively in patients where the MDT decision was to recommend ICA. All had had an ICA to confirm CTCA-MDT findings and, where considered clinically appropriate by the operator, FFR. Definitive management was left to the operator following discussion with the patient. A significant lesion on FFR-CT was defined as <0.8. A significant lesion on FFR was defined as <0.8.
Due to the retrospective nature of clinical care, no ethical approval was sought for this study. Statistical analysis was performed on GraphPad Prism 8.0. For statistical analysis of normally distributed data a Pearson’s correlation coefficient was used with a Spearman’s rank order correlation for non-normally distributed data. CTCA + MDT, FFR-CT and ICA ± FFR were correlated for each coronary artery system. CTCA + MDT and FFR-CT were also correlated with a definitive outcome, that is, whether a PCI was performed or not. CT-FFR was also correlated with the FFR, recorded during ICA, for all vessels.
Table 1. Patient characteristics, risk factors and medication use in patients referred for fractional flow reserve computed tomography (FFR-CT)
End point at four years | |
---|---|
Male | 20/24 (83.3%) |
Female | 4/24 (17%) |
Age, years | 62 ± 9.2 |
Body mass index, kg/m2 | 28 ± 5.1 |
Blood pressure (systolic), mmHg | 132 ± 16.4 |
Blood pressure (diastolic), mmHg | 79 ± 12.7 |
Heart rate (resting), bpm | 56 ± 9.8 |
Risk factors | |
Hypertension | 17/24 (71%) |
Diabetes mellitus | 3/24 (13%) |
Hypercholestrolaemia | 12/24 (50%) |
Smoking (current or previous) | 2/24 (12.5%) |
Family history (IHD) | 9/24 (37.5%) |
Concurrent medication use | |
Statin | 11/24 (46%) |
Aspirin | 9/24 (37.5%) |
Beta blocker | 6/24 (25%) |
ACE inhibitor | 11/24 (46%) |
Thiazide diuretic | 4/24 (17%) |
Calcium channel blocker | 6/24 (25%) |
Data shown as n/N (%) or mean ± standard deviation, as appropriate Key: ACE = angiotensin-converting enzyme; IHD = ischaemic heart disease |
Results
Overall, 13/24 patients (54%) had undergone exercise electrocardiogram (ECG) testing prior to CTCA, with 5/13 (38%) being normal and the remainder being equivocal or abnormal. Two patients did not receive glyceryl trinitrate (GTN) and nine patients did not receive beta blockers prior to the scan due to adequate heart rate control or contraindications. The CTCA scans were performed on either a 64- or 128-detector CT scanner. The mean dose-length product (DLP) was 247 (6.9 mSv using the thoracic conversion factor of 0.028 mSv·mGy-1cm-1). The characteristics of all patients analysed by FFR-CT are detailed in table 1. Of the 24 consecutive patients (n=24/157, 15%) who had FFR-CT, 20 (83%) were accepted for FFR analysis and four (17%) were rejected due to insufficient image quality (due to heavy calcification or large step artefacts).
On CTCA, the median calcium score was 473 (interquartile range [IQR] 259–975) with 88% (n=21/24) over the 50th centile and 38% over the 90th centile. Only one patient had significant left main stem (LMS) disease, which was distal and confluent with a proximal LAD lesion. Overall, we recorded 42 severe stenoses (>70%) of which 17 (41%) were proximal. There were 30 possible flow-limiting stenoses (50–70%), with the remainder (n=96) being mild disease or normal (0–50%). Overall, four patients were CAD-RADS 3 (50–69% maximal stenosis in any vessel), 16 patients were CAD-RADS 4A (70–99% maximal stenosis) and four patients were CAD-RADS 4B (left main >50% or three-vessel disease >70%). All CAD-RADS 3 patients had proximal coronary artery stenosis considered to be of prognostic importance (if flow limiting).
The ICA results ± FFR are described in table 2, where the most significant lesion in each individual coronary system was compared with both CTCA + MDT and FFR-CT. There were 33 apparent critical stenoses (>70%) identified by CTCA + MDT in comparison with 17 identified during ICA. There were 19 PFL at CTCA + MDT with 12 PFL lesions on ICA. There were 20 lesions classified as NFL on CTCA + MDT compared with 43 NFL on ICA. Of the 12 PFL on ICA, nine had FFR performed (75%), of which two were <0.8. Only one NFL lesion on ICA had an FFR performed, which was 0.96. Per vessel correlation showed that CTCA + MDT correlated insignificantly with the FFR-CT and ICA + FFR respectively for all coronary territories. FFR-CT, however, correlated significantly with ICA + FFR for the LAD (r=0.638, p=0.0025) and LCx (r=0.447, p=0.0058) but not the RCA (r=0.350, p=0.130) (table 3). In comparing CTCA + MDT, FFR-CT and definitive outcome, in terms of whether a PCI was performed or not, CTCA + MDT correlated insignificantly with definitive outcome (r=0.378, p=0.07), while FFR-CT was significant (r=0.471, p=0.036).
Table 2. Comparison of computed tomography coronary angiography (CTCA) + multi-disciplinary team (MDT) decision, fractional flow reserve computed tomography (FFR-CT), invasive coronary angiography (ICA) and fractional flow reserve (FFR) for coronary artery systems of all patients referred for FFR-CT
n | Left anterior descending system | Left circumflex system | Right coronary artery system | |||||||||
---|---|---|---|---|---|---|---|---|---|---|---|---|
CT+ MDT |
FFR-CT | ICA | ICA-FFR | CT+ MDT |
FFR-CT | ICA | ICA-FFR | CT+ MDT |
FFR-CT | ICA | ICA-FFR | |
A | PFL | Reject | PFL | 0.77 | CS | Reject | PFL | 0.88 | PFL | Reject | PFL | 0.83 |
B | CS | 0.77 | CS | NP | CS | 0.82 | CS | NP | CS | 0.71 | CS | NP |
C | CS | Reject | NFL | NP | CS | Reject | NFL | NP | CS | Reject | NFL | NP |
D | PFL | 0.86 | NFL | NP | NFL | 0.89 | NFL | NP | NFL | 0.84 | NFL | NP |
E | CS | 0.91 | NFL | NP | NFL | 0.95 | NFL | NP | NFL | 0.91 | NFL | NP |
F | PFL | 0.79 | PFL | 0.78 | NFL | 0.86 | NFL | NP | NFL | 0.88 | NFL | NP |
G | CS | 0.7 | CS | NP | PFL | 0.81 | NFL | NP | NFL | 0.91 | NFL | NP |
H | CS | 0.73 | NFL | 0.82 | NFL | 0.88 | NFL | NP | CS | 0.77 | PFL | 0.88 |
I | NFL | 0.91 | NFL | NP | NFL | 0.93 | NFL | NP | CS | 0.91 | PFL | NP |
J | CS | 0.79 | NFL | NP | PFL | 0.81 | NFL | NP | NFL | 0.97 | NFL | NP |
K | CS | Reject | CS | NP | CS | Reject | CS | NP | CS | Reject | CS | NP |
L | CS | 0.63 | NFL | NP | CS | 0.85 | PFL | NP | PFL | 0.83 | PFL | NP |
M | CS | 0.69 | CS | NP | NFL | 0.92 | NFL | NP | PFL | 0.86 | NFL | 0.9 |
N | CS | 0.77 | NFL | 0.89 | PFL | 0.78 | NFL | NP | PFL | 0.9 | PFL | 0.88 |
O | CS | 0.68 | CS | 0.55 | NFL | 0.92 | NFL | NP | PFL | 0.91 | PFL | 0.88 |
P | PFL | 0.93 | PFL | 0.82 | PFL | 0.96 | NFL | NP | NFL | 0.94 | NFL | NP |
Q | CS | 0.51 | CS | NP | NFL | 0.89 | NFL | NP | NFL | 0.78 | NFL | NP |
R | CS | Reject | CS | 0.75 | CS | Reject | PFL | 0.84 | CS | Reject | NFL | NP |
S | CS | 0.74 | CS | 0.76 | PFL | 0.83 | NFL | NP | CS | 0.78 | NFL | NP |
T | PFL | 0.83 | NFL | 0.96 | PFL | 0.82 | NFL | NP | NFL | 0.95 | NFL | NP |
U | CS | 0.6 | CS | 0.55 | NFL | 0.88 | NFL | NP | NFL | 0.9 | NFL | NP |
V | CS | 0.62 | CS | NP | PFL | 0.82 | NFL | NP | PFL | 0.79 | NFL | NP |
W | CS | <0.5 | CS | NP | CS | 0.62 | CS | NP | PFL | 0.74 | NFL | NP |
X | CS | 0.72 | CS | 0.65 | NFL | 0.81 | NFL | NP | CS | 0.81 | NFL | NP |
Key: CS = criticial stenosis (>70% stenosis); NFL = not flow limiting <50%; PFL = potentially flow limiting stenosis (50–69%); Reject = rejected FFR-CT request by HeartFlow due to CTCA image quality |
Table 3. Correlation matrix of computed tomography (CT) coronary angiogram with MDT reporting (CTCA+MDT), CT Fractional Flow Reserve (FFR-CT) and invasive coronary angiogram ± fractional flow reserve (ICA±FFR) for the left anterior descending system (LAD), right coronary artery (RCA) and circumflex (Cx)
CTCA+MDT | FFR-CT | ||
---|---|---|---|
LAD | FFR-CT | 0.397 | |
ICA±FFR | 0.246 | 0.638** | |
RCA | FFR-CT | 0.373 | |
ICA±FFR | 0.234 | 0.350 | |
Cx | FFR-CT | 0.309 | |
ICA±FFR | 0.302 | 0.447* | |
* Denotes p<0.05, ** denotes p<0.01 |
In five cases (21%, 5/24), the FFR-CT was >0.8 but the clinician proceeded to ICA. In three patients, the MDT consensus was that the coronary lesion was not flow limiting on CTCA but there was significant clinical suspicion for the clinician to proceed with ICA. In two patients, the MDT consideration was that the coronary lesion on CTCA was severe but the FFR-CT was >0.8 and the definitive outcome was OMT. In a further instance (patient F, table 2), FFR-CT indicated a potentially more significant stenosis in the LAD than was apparent on CTCA alone. While the definitive outcome was for OMT, the patient later re-presented with recurrent symptoms and underwent revascularisation. FFR-CT of 60 coronary artery vessels (83%, 60/72) (mean FFR-CT ratio 0.82 ± 0.10) compared well with FFR performed on 18 coronary vessels (mean 0.80 ± 0.11) with a correlation coefficient of 0.758 (p=0.0013) (figure 1).
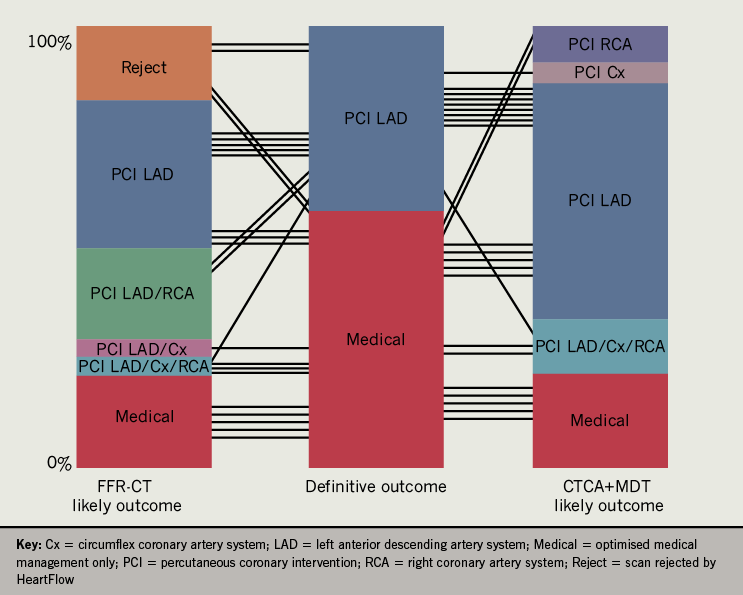
Discussion
The main finding of these data is that ‘real-world’ FFR-CT, if utilised in patients recommended for an ICA, has the potential to alter management in 21% by either confirming that a moderate lesion (50–70%) on CTCA is non-flow limiting or by altering the MDT consensus that an apparently critical stenosis on CTCA was physiologically insignificant. In a further instance (patient F), FFR-CT indicated a potentially more significant stenosis in the LAD than was apparent on CTCA alone, which is of interest since the patient re-presented with recurrent symptoms and underwent revascularisation.
FFR-CT has been shown in large trials to be superior to CTCA alone in deferring the requirement for ICA,14,15,19,29,30 with the potential to change interpretations/recommendations in approximately a quarter of CTCA scans.31 Investigators of the ADVANCE (Assessing Diagnostic Value of Non-invasive FFRCT in Coronary Care) registry assessed the FFR-CT results on subsequent management decisions in 5,083 subjects who had 30–90% stenosis on CTCA (of which 4,737 underwent FFR-CT) at 38 international sites.20 Approximately two thirds of subjects had a change in management following FFR-CT results.
The FFR-CT RIPCORD (Does Routine Pressure Wire Assessment Influence Management Strategy at Coronary Angiography for Diagnosis of Chest Pain?) study assessed 200 CTCA scans (from the NXT study) where the addition of FFR-CT to CCTA changed the management plan of 36% of patients with a 5% decrease in PCI.30 Retrospective analysis of patients having CCTA scans in the PROMISE (PROspective Multicenter Imaging Study for Evaluation of Chest Pain) trial using adjunctive FFR-CT, 31% discordance was observed between CCTA and FFR-CT.19
Nørgaard et al.32 investigated real-world patients presenting with new-onset chest pain to establish whether management would be changed by addition of FFR-CT. FFR-CT was performed in 185 patients with 31% having a FFR-CT measurement of <0.80 and 9% proceeding to ICA based on this result. There was correct correlation in 73% of patients and 70% of vessels when compared with FFR. There were no significant adverse cardiac outcomes in the 123 (66%) patients who were diverted from undergoing ICA based on their FFR-CT results.
In a study analysing 82 patients from the DeFACTO (Determination of Fractional Flow Reserve by Anatomic Computed Tomographic Angiography) study, FFR-CT performed significantly better than CTCA, with nearly one quarter of intermediate lesions being associated with ischaemia. In this instance, intermediate stenosis were defined as 30–69%,17 although the authors reported that 22% of milder lesions, in the range of 30–49% stenosis, were causal of ischaemia.
In a subanalysis of DISCOVER-FLOW (Diagnosis of Ischemia-Causing Stenoses Obtained Via Noninvasive Fractional Flow Reserve), intermediate lesions were defined as 40–69%, there was a 25% improvement in diagnostic accuracy with FFR-CT. While this evidence would suggest that FFR-CT is well utilised in the assessment of intermediate lesions, FFR-CT can also alter the requirements for ICA even when restricted to being used in severe lesions (>70%).19
Projections indicate FFR-CT processing may be required in 20–25% of patients having CCTA.16 We presently recommend 24% of patients (n=37/157) for ICA on the basis of MDT discussion.27 Of these, 65% (n=24/37) have no obstructive disease. The adoption of FFR-CT may, therefore, reduce ICA in our trust by 65% if we utilised FFR-CT for this group. While the use of FFR-CT is free to NHS hospital trusts, being currently paid for by NHS England, arguably the cost-effectiveness of FFR-CT would reduce significantly if utilised for all patients who underwent a CTCA. The utilisation of FFR-CT may, therefore, be best served to investigate coronary stenosis on CTCA or prognostically important PFL lesions. Presently, we do not know what NHS England are reimbursing HeartFlow for FFR-CT. As technology progresses and other commercial vendors evidence their algorithms, FFR-CT may be used routinely in all CTCA scans.
Four patients (17%) in our study were rejected on the basis of their scans being of inadequate quality, which is similar to US real-world data showing an acceptance rate of 21%. It is worth mentioning, however, that our scans had already been pre-screened, from a technology perspective, at the time of the MDT for suitability for FFR-CT. The majority of our scans were performed on 64-detector CT scanners, so we would anticipate that the suitability of CTCA scans for FFR-CT analysis would increase further with adoption of more advanced scanners. By way of comparison, our rejection rates were larger than rates reported in the NXT (Analysis of Coronary Blood Flow Using CT Angiography: Next Steps) trial (13%),15 PLATFORM (Prospective Longitudinal Trial of FFRCT: Outcome and Resource Impacts) trial (12%),29 and much larger than the 3% reported in the analysis of the ADVANCE registry, although concerns regarding selection bias have been raised with respect to this.33
This study is limited significantly by being retrospective and a small data set of only 24 FFR-CTs with incomplete FFR data. As there was no control group, one cannot ascertain what happened to the patients who did not undergo an FFR-CT nor ICA.27 There were no major adverse cardiac events (MACE) events occurring in the 87% (n=137/157) who did not undergo FFR-CT during the relatively long follow-up period, albeit in small numbers. To that end, definitive conclusions cannot be drawn from these data. We also did not perform any cost-saving analysis of FFR-CT use in our centre on this cohort. The strength of our investigation, by reporting a consecutive series of patients referred for ICA, is to show where FFR-CT might fit into a complex diagnostic pathway, so informing other non-PCI NHS trusts and those involved in healthcare commissioning.
Conclusion
FFR-CT has the potential to further improve diagnostic accuracy to a district general hospital diagnostic CTCA service for investigating patients recommended for ICA, including those with prognostically important proximal PFL disease.
Key messages
- Fractional flow reserve computed tomography (FFR-CT, HeartFlow) is supported by the National Institute of Health and Care Excellence (NICE) due to the potential reduction in invasive coronary angiograms showing non-obstructive coronary artery disease and subsequent cost-savings
- Prognostically an FFR-CT of <0.8 is associated with a significantly higher risk of myocardial infarction, death and revascularisation
- FFR-CT, when utilised to assess moderate lesions of prognostic importance or severe disease, as identified on computed tomography coronary angiography (CTCA), has the potential to alter management in approximately one-fifth of patients, in addition to multi-disciplinary reporting
Conflicts of interest
MH and EL have shares in Virtual Cath Lab – a private CT coronary angiography company.
Funding
The project was unfunded.
Study approval
Due to the retrospective nature of clinical care, no ethical approval was sought for this study.
References
1. National Institute for Health and Care Excellence. Chest pain of recent onset: assessment and diagnosis. CG95. London: NICE, 2016. Available from: https://nice.org.uk/guidance/cg95
2. Meijboom WB, Meijs MF, Schuijf JD et al. Diagnostic accuracy of 64-slice computed tomography coronary angiography: a prospective, multicenter, multivendor study. J Am Coll Cardiol 2008;52:2135–44. https://doi.org/10.1016/j.jacc.2008.08.058
3. Meijboom WB, Van Mieghem CAG, van Pelt N et al. Comprehensive assessment of coronary artery stenoses: computed tomography coronary angiography versus conventional coronary angiography and correlation with fractional flow reserve in patients with stable angina. J Am Coll Cardiol 2008;52:636–43. https://doi.org/10.1016/j.jacc.2008.05.024
4. Douglas PS, De Bruyne B, Pontone G et al. 1-year outcomes of FFRCT-guided care in patients with suspected coronary disease: the PLATFORM study. J Am Coll Cardiol 2016;68:435–45. https://doi.org/10.1016/j.jacc.2016.05.057
5. SCOT-HEART Investigators. CT coronary angiography in patients with suspected angina due to coronary heart disease (SCOT-HEART): an open-label, parallel-group, multicentre trial. Lancet 2015;385:2383–91. https://doi.org/10.1016/S0140-6736(15)60291-4
6. Douglas PS, Hoffmann U, Patel MR et al. Outcomes of anatomical versus functional testing for coronary artery disease. N Engl J Med 2015;372:1291–300. https://doi.org/10.1056/NEJMoa1415516
7. Foy AJ, Dhruva SS, Peterson B, Mandrola JM, Morgan DJ, Redberg RF. Coronary computed tomography angiography vs functional stress testing for patients with suspected coronary artery disease: a systematic review and meta-analysis. JAMA Intern Med 2017;177:1623–31. https://doi.org/10.1001/jamainternmed.2017.4772
8. Nielsen LH, Ortner N, Nørgaard BL et al. The diagnostic accuracy and outcomes after coronary computed tomography angiography vs. conventional functional testing in patients with stable angina pectoris: a systematic review and meta-analysis. Eur Heart J Cardiovasc Imaging 2014;15:961–71. https://doi.org/10.1093/ehjci/jeu027
9. Goldstein JA, Gallagher MJ, O’Neill WW et al. A randomized controlled trial of multi-slice coronary computed tomography for evaluation of acute chest pain. J Am Coll Cardiol 2007;49:863–71. https://doi.org/10.1016/j.jacc.2006.08.064
10. Tonino P, De Bruyne B, Pijls NH et al. Fractional flow reserve versus angiography for guiding percutaneous coronary intervention. N Engl J Med 2009;360:1045–57. https://doi.org/10.1056/NEJMoa0807611
11. Ihdayhid AR, Sapontis J. The fractional flow reserve grey zone: a blueprint for the future of coronary revascularisation. Heart 2020;106:714–15. https://doi.org/10.1136/heartjnl-2019-316435
12. Nicol ED, Nørgaard BL, Blanke P et al. The future of cardiovascular computed tomography: advanced analytics and clinical insights. JACC Cardiovasc Imaging 2019;12:1058–72. https://doi.org/10.1016/j.jcmg.2018.02.001
13. Koo B-K, Erglis A, Doh J-H et al. Diagnosis of ischemia-causing coronary stenoses by noninvasive fractional flow reserve computed from coronary computed tomographic angiograms. Results from the prospective multicenter DISCOVER-FLOW (Diagnosis of Ischemia-Causing Stenoses Obtained Via Noninvasive Fractional Flow Reserve) study. J Am Coll Cardiol 2011;58:1989–97. https://doi.org/10.1016/j.jacc.2011.06.066
14. Min JK, Leipsic J, Pencina MJ et al. Diagnostic accuracy of fractional flow reserve from anatomic CT angiography. JAMA 2012;308:1237–45. https://doi.org/10.1001/2012.jama.11274
15. Nørgaard BL, Leipsic J, Gaur S et al. Diagnostic performance of noninvasive fractional flow reserve derived from coronary computed tomography angiography in suspected coronary artery disease: the NXT trial (Analysis of Coronary Blood Flow Using CT Angiography: Next Steps). J Am Coll Cardiol 2014;63:1145–55. https://doi.org/10.1016/j.jacc.2013.11.043
16. Asher A, Singhal A, Thornton G, Wragg A, Davies C. FFRCT derived from computed tomography angiography: the experience in the UK. Expert Rev Cardiovasc Ther 2018;16:919–29. https://doi.org/10.1080/14779072.2018.1538786
17. Nakazato R, Park HB, Berman DS et al. Noninvasive fractional flow reserve derived from computed tomography angiography for coronary lesions of intermediate stenosis severity results from the DeFACTO study. Circ Cardiovasc Imaging 2013;6:881–9. https://doi.org/10.1161/CIRCIMAGING.113.000297
18. Agasthi P, Kanmanthareddy A, Khalil C et al. Comparison of computed tomography derived fractional flow reserve to invasive fractional flow reserve in diagnosis of functional coronary stenosis: a meta-analysis. Sci Rep 2018;8:11535. https://doi.org/10.1038/s41598-018-29910-9
19. Lu MT, Ferencik M, Roberts RS et al. Noninvasive FFR derived from coronary CT angiography: management and outcomes in the PROMISE trial. JACC Cardiovasc Imaging 2017;10:1350–8. https://doi.org/10.1016/j.jcmg.2016.11.024
20. Fairbairn TA, Nieman K, Akasaka T et al. Real-world clinical utility and impact on clinical decision-making of coronary computed tomography angiography-derived fractional flow reserve: lessons from the ADVANCE Registry. Eur Heart J 2018;39:3701–11. https://doi.org/10.1093/eurheartj/ehy530
21. Nørgaard BL, Terkelsen CJ, Mathiassen ON et al. Coronary CT angiographic and flow reserve-guided management of patients with stable ischemic heart disease. J Am Coll Cardiol 2018;72:2123–34. https://doi.org/10.1016/j.jacc.2018.07.043
22. Renker M, Schoepf UJ, Wang R et al. Comparison of diagnostic value of a novel noninvasive coronary computed tomography angiography method versus standard coronary angiography for assessing fractional flow reserve. Am J Cardiol 2014;114:1303–08. https://doi.org/10.1016/j.amjcard.2014.07.064
23. Coenen A, Lubbers MM, Kurata A et al. Fractional flow reserve computed from noninvasive CT angiography data: diagnostic performance of an on-site clinician-operated computational fluid dynamics algorithm. Radiology 2015;274:674–83. https://doi.org/10.1148/radiol.14140992
24. Ko BS, Cameron JD, Munnur RK et al. Noninvasive CT-derived FFR based on structural and fluid analysis: a comparison with invasive FFR for detection of functionally significant stenosis. JACC Cardiovasc Imaging 2017;10:663–73. https://doi.org/10.1016/j.jcmg.2016.07.005
25. National Institute for Health and Care Excellence (NICE). HeartFlow FFRCT for estimating fractional flow reserve from coronary CT angiography. London: NICE, 2017. Available from: https://www.nice.org.uk/guidance/mtg32/chapter/2-The-technology#description-of-the-technology
26. Rajani R, Webb J, Marciniak A, Preston R. Comparative efficacy testing – fractional flow reserve by coronary computed tomography for the evaluation of patients with stable chest pain. Int J Cardiol 2015;183:173–7. https://doi.org/10.1016/j.ijcard.2015.01.035
27. Parsons IT, Bannister C, Badelek J et al. The HASTE protocol: a standardised CT coronary angiography service operated from a district general hospital. Open Heart 2018;5:e000817. https://doi.org/10.1136/openhrt-2018-000817
28. Cury RC, Abbara S, Achenbach S et al. Coronary Artery Disease – Reporting and Data System (CAD-RADS): an Expert Consensus Document of SCCT, ACR and NASCI: Endorsed by the ACC. JACC Cardiovasc Imaging 2016;9:1099–113. https://doi.org/10.1016/j.jcmg.2016.05.005
29. Douglas PS, Pontone G, Hlatky MA et al. Clinical outcomes of fractional flow reserve by computed tomographic angiography-guided diagnostic strategies vs. usual care in patients with suspected coronary artery disease: the prospective longitudinal trial of FFR(CT): outcome and resource impacts study. Eur Heart J 2015;36:3359–67. https://doi.org/10.1093/eurheartj/ehv444
30. Curzen NP, Nolan J, Zaman AG, Norgaard BL, Rajani R. Does the routine availability of CT-derived FFR influence management of patients with stable chest pain compared to CT angiography alone? The FFRCT RIPCORD study. JACC Cardiovasc Imaging 2016;9:1188–94. https://doi.org/10.1016/j.jcmg.2015.12.026
31. Fares A, Alaiti MA, Alkhalil A et al. Real world utilization of computed tomography derived fractional flow reserve: single center experience in the United States. Cardiovasc Revasc Med 2019;20:1043–7. https://doi.org/10.1016/j.carrev.2019.01.019
32. Nørgaard BL, Hjort J, Gaur S et al. Clinical use of coronary CTA-derived FFR for decision-making in stable CAD. JACC Cardiovasc Imaging 2017;10:541–50. https://doi.org/10.1016/j.jcmg.2015.11.025
33. Villines TC. Can CT-derived FFR better inform clinical decision-making and improve outcomes in stable ischaemic heart disease? Eur Heart J 2018;39:3712–14. https://doi.org/10.1093/eurheartj/ehy559