In this series of articles looking at contemporary ways to image atherosclerosis, we have covered the main techniques used in the UK as diagnostic tools and research modalities. For this final article, I have chosen to concentrate on new technologies that show promise for the future.
Introduction
As a way of moving on from our previous articles, I have devised a summary table (table 1) looking at what can or cannot be provided by the existing techniques and what may be provided by the newer techniques that will be discussed briefly in this article.

Future plaque imaging techniques
Raman spectroscopy

Raman spectroscopy is an established analytical technique that is widely utilised throughout the world. Over the last decade, a Raman spectroscopy catheter system has been built that provides chemical contour maps of the full luminal area of a human coronary artery. These contour maps of chemical concentrations within the arterial wall appear to agree closely with histology findings, this may allow the chemical diagnosis of a thin-cap fibroatheroma.
Atherosclerotic plaque composition clearly plays a large role in determining whether a given lesion will rupture and cause an acceleration of clinical symptoms.1,2 Specifically, the progression and regression of certain plaques appears to be related to the amount and type of lipid that has accumulated within an artery. The relative amount of cholesterol increase in ruptured plaques, compared with less advanced plaques also plays a significant role.3
Optical spectroscopy appears to show promise in providing detailed arterial compositional information, as it has been used to identify unknown substances in countless applications. Starting in the 1980s, researchers studied different forms of optical spectroscopy, as they found that molecular vibrations are primarily determined by the atomic configuration within any given molecule, with each having its own particular spectrum.4
Two main spectroscopic technologies that can measure molecular internal structure are undergoing development: Raman spectroscopy and near infrared spectroscopy (NIRS). Compared with Raman spectroscopy, NIRS apparently can have a more intense signal, but is only capable of detecting the general biochemical category of lipid.5 However, Raman spectroscopy can detect lipid categories and subcategories, such as triglycerides and many other compounds. It could potentially provide insight into the entire chemical composition of the plaque.6 The ability of Raman spectroscopy to specifically identify and quantify the types of lipids in the arterial wall may also increase the ability to treat a patient individually.7 These techniques are now being combined with intravascular ultrasound (IVUS) to allow invasive plaque imaging with chemical composition (figures 1 and 2).

NIRS-IVUS
‘Lipiscan™’ IVUS has now been cleared for sale in the USA and uses NIRS to detect areas of lipid core providing a ‘chemogram™’ linked to the IVUS pullback. This NIRS is based upon the different absorption of light at different wavelengths by different chemicals. This allows co-registration of coronary anatomy and true lipid composition. This technique not only advances our knowledge of atherosclerosis but appears to show promise in determining which patients may sustain a peri-procedural myocardial infarction.8 It may also become useful in judging the length of stent to place in order to prevent landing edges into unstable lipid cores.
Thermography
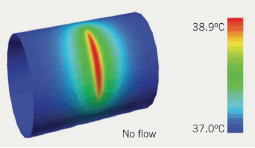
Although not widely used in the UK clinically, it is known that temperature measurements can be used to detect local inflammatory processes in the arterial wall (figure 3). The thermistor on a standard thermography catheter is capable of measuring temperatures superficially with an accuracy around 0.1°C. Casscells et al.9 showed in freshly obtained carotid endarterectomy specimens a temperature rise up to 2.2°C in macrophage-rich areas. In that study, a significant correlation was observed between macrophage density and local temperature. Moreover, one of the first studies to assess local thermal heterogeneity, in the coronary artery, reported higher temperatures in lesions of patients suffering from unstable angina and myocardial infarction compared with lesions studied in patients with stable angina.10 Although plaque temperature may give us a clue to which plaques are ‘hot’, it clearly needs co-registration with anatomy to be of any relevance and may remain confined to the research world until it is combined in a multi-modal catheter.
Palpography

Determining the biomechanical stress being applied to a plaque with every pulse of blood in the coronary artery is understandably very important in relation to the risk of plaque rupture and the aetiology of why plaques develop and progress in the first instance. The local, mechanical tissue properties at a defined point appear to show that soft tissue (lipid-rich) components will deform more than hard components (fibrous-calcified). The technique was validated in vitro using diseased human coronary and femoral arteries. A high-strain region (yellow signal) at the lumen vessel wall boundary was originally quoted to have 88% sensitivity and 89% specificity for identifying unstable plaques (figure 4).11 Furthermore, in the Integrated Biomarker and Imaging Study (IBIS) it was shown that the density of strain spots above decreased significantly in acute coronary syndrome (ACS) patients having standard care.11 In IBIS 2, it was shown that patients with vulnerable plaques that were treated with darapladib showed a significant decrease in strain values after 12 months.12 However, in the recent Providing Regional Observations to Study Predictors of Events Coronary Tree (PROSPECT) analysis13 it seems (from personal correspondence with the trial sponsors) that palpography did not provide any useful insight into the plaques eventually leading to coronary events. The final results from PROSPECT have just been published and palpography does not feature in the main paper.13
Near infrared fluorescence

Near infrared fluorescence (NIRF) may well be the future of invasive molecular imaging (figure 5). The development of a coronary artery-compatible NIRF sensing catheter coupled with a high-sensitivity protease activatable agent has enabled the real-time sensing of plaque inflammation through blood. Anticipated development of third-generation ‘multi-modality’ NIRF catheters is poised to enable integrated molecular and architectural assessment of coronary plaques in the cath lab.14 The availability of clinical coronary NIRF catheters with approved NIRF imaging agents will provide new insights into atherosclerosis biology in vivo. This may lead to a true understanding of an individual plaque’s risk of inducing myocardial infarction based on its underlying biological profile. It will also allow the assessment of novel biologics designed to attenuate inflammation in coronary atherosclerotic plaques and potentially prevent their rupture.
The inflammatory cascade has yielded a number of high-sensitivity molecular imaging targets. Macrophages, cysteine proteases and matrix metalloproteinases that destabilise the fibrous cap overlying plaques, are all potentially translatable NIRF imaging targets to detect plaque inflammation. In addition, leukocyte adhesion molecules, e.g. vascular cell adhesion molecule (VCAM), are also attractive imaging targets, due to their ability to report on early plaque inflammation.15 The fine details of this technique are beyond the scope of this mini-review, however, I would definitely say “watch this space”.
Conclusions
It is clear that future invasive catheter developments will require technological advances to allow multi-modality catheters. The ability to switch from a whole vessel and plaque IVUS view to a near-field, high-resolution optical coherence tomography (OCT) view would be highly desirable. These catheters may then incorporate functionality switches, enabling them to provide co-registration of virtual histology (VH)/NIRS/NIRF/Raman spectroscopy/thermography and palpography. However, advances in radiolabelling techniques with positron emission tomography (PET), single photon emission tomography (SPECT) and molecular agents targeting plaque components, may see non-invasive imaging via computed tomography (CT) and magnetic resonance imaging (MRI) provide the future gold standard of plaque imaging.
Although the struggle to find the ultimate investigational strategy to prevent and treat coronary artery disease continues, we remain stuck at a stage where the need to find a ‘plaque holy grail’ is overshadowed by the task of proving that it will ultimately translate into improved patient outcomes. I fear there is a long road ahead.
Conflict of interest
None declared.
Editors’ note
This is the final article in our imaging series. Previous articles in this series have covered magnetic resonance imaging, computed tomography, optical coherence tomography and intravascular ultrasound derived virtual histology. These are all available online at
www.bjcardio.co.uk
References
1. Libby P. Molecular bases of the acute coronary syndromes. Circulation 1995;91:2844–50.
2. Virmani R, Burke A, Farb A et al. Pathology of the vulnerable plaque. In: Waksman R, Serruys PW, eds. Handbook of the Vulnerable Plaque. New York: Martin Dunitz, 2004;33–48.
3. Felton CV, Crook D, Davies MJ et al. Relation of plaque lipid composition and morphology to the stability of human aortic plaques. Arterioscler Thromb Vasc Biol 1997;17:1337–45.
4. Hammes GG. Spectroscopy for the Biological Sciences. New Jersey: Wiley, 2005.
5. Gardner CM, Tan H, Hull E et al. Detection of lipid core coronary plaques in autopsy specimens with a novel catheter-based near-infrared spectroscopy system. JACC Cardiovasc Imaging 2008;1:638–48.
6. Buschman HP, Marple ET, Wach ML et al. In vivo determination of the molecular composition of artery wall by intravascular Raman spectroscopy. Anal Chem 2000;72:3771–5.
7. Römer TJ, Brennan JF, Fitzmaurice M et al. Histopathology of human coronary artery atherosclerosis by quantifying its chemical composition with Raman spectroscopy. Circulation 1998;97:878–85.
8. Saeed B, Banerjee S, Brilakis ES. Slow flow after stenting of a coronary lesion with a large lipid core plaque detected by near-infrared spectroscopy. Eurointervention 2010;6:545.
9. Casscells W, Hathorn B, David M et al. Thermal detection of cellular infiltrates in living atherosclerotic plaques: possible implications for plaque rupture and thrombosis. Lancet 1996;347:1447–9.
10. Stefanadis C, Diamantopoulos L, Vlachopoulos C et al. Thermal heterogeneity within human atherosclerotic coronary arteries detected in vivo. Circulation 1999;99:1965–71.
11. Van Mieghem CA, McFadden EP, de Feyter PJ et al. Noninvasive detection of subclinical coronary atherosclerosis coupled with assessment of changes in plaque characteristics using novel invasive imaging modalities: the Integrated Biomarker and Imaging Study (IBIS). J Am Coll Cardiol. 2006;47:1134–42.
12. Serruys PW, García-García HM, Buszman P et al.; for the Integrated Biomarker and Imaging Study-2 Investigators. Effects of the direct lipoprotein-associated phospholipase A2 inhibitor darapladib on human coronary atherosclerotic plaque. Circulation 2008;118:1172–82.
13. Stone GW, Maehara A, Lansky AJ et al. A prospective natural history study of coronary atherosclerosis.
N Engl J Med 2011;364:226–35.
14. Jaffer FA, Vinegoni C, John MC et al. Real-time catheter molecular sensing of inflammation in proteolytically active atherosclerosis. Circulation 2008;118:1802–09.
15. Chen J, Tung CH, Mahmood U et al. In vivo imaging of proteolytic activity in atherosclerosis. Circulation 2002;105:2766–71.