Introduction
Device therapies, including cardiac resynchronisation therapy (CRT) pacemakers and implantable cardioverter-defibrillators (ICDs), are established treatment options for patients with symptomatic heart failure (HF) due to reduced ejection fraction (HFrEF) despite optimal medical therapy (OMT).
Other treatments have been trialled and are recommended in select cases, such as ultrafiltration; and newer therapies such as gene therapy, may have a role in future. Here, we will review these other invasive non-surgical treatment options. There are no device therapies approved for use in patients with HF and a left ventricular ejection fraction (LVEF) >35%.
Implantable devices

CRT
What is CRT?1,2
CRT is a useful adjunct to optimal medical therapy in patients with left ventricular systolic dysfunction (LVSD) and an ejection fraction (EF) ≤35%. In clinical trials, CRT has proven to reduce both mortality and HF hospitalisation rates. It also improves symptoms, functional state and quality of life (QoL).
Many patients with LVSD have a delay in the electrical conduction through the heart. The electrical conduction delay is identified on a 12-lead electrocardiogram (ECG) by a widened QRS complex (>120 ms), often meeting criteria for a bundle branch block (BBB). Over 30% of patients with LVSD have left bundle branch block (LBBB) in comparison to <1% of the general population (figure 1). The rate of new LBBBs in patients with HF is around 10% per year. Atrioventricular (AV) nodal disease, particularly first-degree heart block, is also common in LVSD.

Pacing via CRT uses the coronary sinus as an access route to a pacing position on the left ventricle (LV) via a vein draining the left lateral wall of the heart. The hypothesis is that CRT, which paces the right ventricular (RV) apex (or septum) LV free wall simultaneously, corrects interventricular dyssynchrony (and shortens the QRS duration). It is commonly assumed that this translates to more co-ordinated LV systolic contraction.
Despite the success of CRT in clinical practice, it is not clear how CRT mediates its benefits. Much discussion has centred on the mechanical consequences of a prolonged QRS duration, particularly:
- late LV contraction relative to RV contraction – interventricular dyssynchrony
- unco-ordinated LV systolic contraction – intraventricular dyssynchrony.
Mechanical dyssynchrony
The mechanisms underlying the benefit of CRT are not clear. There is no convincing evidence that any one of several measures of mechanical dyssynchrony is associated with the beneficial effects of CRT, and the latest (2014) National Institute for Health and Clinical Excellence (NICE) guidance on CRT has removed any reference to measuring mechanical dyssynchrony on echocardiography as part of patient selection.3
QRS morphology, rather than duration, may be the important factor for predicting response and survival following CRT device implantation; LBBB is associated with improved survival following CRT device implantation compared to patients with non-LBBB QRS morphology.4
However, it may be that a major part of the benefit of CRT is simply to shorten the AV delay. Old studies in patients with HF and a prolonged PR interval showed that sequential AV pacing reduced mitral regurgitation and could improve some aspects of myocardial function. It may be that CRT (by pacing both ventricles) shortens the AV delay, whilst avoiding the potentially harmful effects of RV apical pacing alone.5
Some patients with LVSD are also at high risk of ventricular arrhythmias and CRT can be combined with defibrillation therapy (CRT-D) (ICDs are covered below). The pacing element of the device alone (CRT-P) is used in patients for whom defibrillation therapy is not appropriate.3
Benefits of CRT
- reduced mortality rate
- reduced HF hospitalisation rate
- improved New York Heart Association (NYHA) class
- improved six-minute walk test distance
- improved cardiopulmonary exercise testing
- improved QoL questionnaire scores in over two-thirds of the patients undergoing the procedure
- increased systolic arterial pressure
- improved myocardial efficiency – improved LV function without increased LV oxygen consumption
- reduced LV size and mass, and reduction in severity of mitral regurgitation
- in some patients, ‘super-responders’, LV systolic function may ‘normalise’.
Box 1. Predictors of ‘response’ to CRT
QRS complex ≥150 ms |
LBBB morphology |
Non-ischaemic cardiomyopathy |
Female sex |
Sinus rhythm |
Key: CRT = cardiac resynchronisation therapy; LBBB = left bundle branch block |
However, around 20–30% of patients treated with CRT do not appear to benefit in terms of either symptomatic improvement or improvement in LV size or function.6
There is no universally agreed definition of ‘response’. It is not at all clear whether a ‘non-responder’ might actually have deteriorated without the CRT (and hence ‘response’ might be ‘failure to deteriorate’), and it is not clear if a lack of clinical response translates into a lack of mortality benefit from CRT.
Patient selection for CRT
CRT is recommended for patients with severe LVSD (LVEF ≤35%) and BBB morphology on ECG who remain symptomatic despite OMT.3,7 There is no randomised controlled trial (RCT) evidence to support the use of CRT in patients with narrow QRS complexes (QRS <120 ms). It is also fundamental to note that CRT is not a replacement for medication.
CRT in the guidelines
NICE provided guidance in 2014 on the use of CRT in patients with severe LVSD based on NYHA class, QRS duration and presence of a LBBB.3 In line with international guidance, NICE no longer stipulates that patients should be in sinus rhythm to be considered for CRT (see summary at the end of this module).
NICE recommends CRT for patients who meet the following criteria3:
- QRS >150 ms, regardless of BBB morphology or symptoms
- QRS 120–149 ms with LBBB morphology and NYHA II symptoms or worse
- QRS 120–149 ms with non-LBBB morphology and NYHA class IV symptoms.
If a patient with a simple pacemaker is receiving a substantial amount of RV pacing and develops worsening HF symptoms despite OMT, then an upgrade to CRT is recommended, regardless of LVEF.7 |
European Society of Cardiology (ESC) HF guidelines recommend a similar approach but broaden the selection criteria for symptomatic patients with a QRS duration of 130–149 ms and non-LBBB morphology to include those with NYHA II and III symptoms.7
The 2021 ESC guidelines also recommend CRT over RV pacing for patients with heart failure with reduced ejection fraction (HFrEF) who have an indication for ventricular pacing and a high-degree AV block, regardless of LVEF or symptoms.3,7 HFrEF is defined as a LVEF of 40% or less.
How do you implant a CRT device?
A common misconception is that insertion of a CRT device is similar to that of a pacemaker used to treat bradycardia. Whilst some similarities exist, there are significant and important differences in terms of the procedure itself and potential adverse effects.
A CRT device is commonly implanted under local anaesthetic and takes one to three hours. The patient may be in hospital overnight.
CRT devices consist of the following:
- small battery-powered generator (about the size of a match box) containing circuitry and a battery
- leads (wires) that are placed in the heart using X-ray guidance.
The leads stay in contact with the myocardium at one end, while the other end is connected to the generator.
Insertion of the right atrial and RV leads is performed in the same way as for a pacemaker used to treat bradycardia.
The significant difference for a CRT device is placement of a lead to allow pacing of the LV. In general, a lead cannot be positioned within the LV due to the thromboembolic risk associated with a foreign body inside the systemic circulation.
The LV lead is typically placed transvenously via the coronary sinus (CS) to lie on the epicardial (outside) surface of the left heart.
The CS lies in the left AV groove, collects blood from the myocardium and drains into the right atrium through the Thebesian valve.
Step 1 |
|
Step 2 |
Step 3
LV pacing requires placement of a pacing lead down a suitable tributary of the CS, preferably in a posterolateral location. The lead may then be advanced over an angioplasty guidewire into the appropriate position (figures 4 and 5).
A common problem with CRT is inadvertent phrenic nerve stimulation, which results in diaphragmatic contraction at the programmed pacing rate – a particularly unpleasant side effect. This has been overcome, to a certain degree, by the development of LV pacing leads with multiple pacing points (electrodes) that can be switched on or off electronically without the need to reposition the lead. Step 4 |
![]()
|
Adverse events related to CRT device implantation8
The majority of adverse events during CRT device implantation relate to LV lead placement and include:
- failure to implant LV lead (4.5–8.5%)
- coronary sinus dissection (0.5–2.1%)
- cardiac perforation/tamponade (0.3–2.1%)
- extracardiac stimulation/phrenic nerve stimulation (0.8–4%)
- LV lead dislodgement (0.4%)
- death (0.01–0.03%).
Monitoring of CRT7
Following successful implantation, the CRT device needs to be programmed to ensure as near to 100% pacing as possible. The P wave-to-pacing stimulus interval has to be short to prevent normal LV activation via the AV node.
The device should be checked in the pacing clinic for levels of biventricular pacing (aiming for 100%), arrhythmia detection and pacing variables at set intervals.
Evidence
It is important to note that the major CRT RCTs have recruited patients fulfilling select inclusion criteria (LVEF ≤35%, mainly in NYHA class II–III, in sinus rhythm with LBBB and a QRS duration >150 ms). Evidence is therefore strongest in this patient group.
The COMPANION (Comparison of Medical Therapy, Pacing, and Defibrillation in Heart Failure) trial (n=1,520) showed a relative risk reduction (RRR) in death of 24% with a CRT-P (p=0.059) and 36% with CRT-D (p=0.003) compared to medical therapy.9
In a similar patient population, the CARE-HF (Cardiac Resynchronisation — Heart Failure) trial (n=813) compared CRT-P to medical therapy.10 The primary end point of time to death from any cause or hospitalisation for a major cardiovascular event occurred in 39% of patients receiving CRT-P compared to 55% of patients receiving medical therapy alone, with a significant reduction in mortality in the CRT arm versus medical therapy alone arm (20% vs. 30%, respectively; p<0.002).10
Both the MADIT-CRT (Multicenter Automatic Defibrillator Implantation Trial with Cardiac Resynchronisation Therapy) (n=1,820; NYHA I, 15%; NYHA II, 85%)11 and RAFT (Resynchronisation–Defibrillation for Ambulatory Heart Failure Trial) (n=1,798; NYHA class II, 80%, NYHA III, 20%)12 trials confirmed a similar reduction in HF events and survival benefit for CRT in patients with mild symptoms.
In both trials, more than half of the patients had HF due to ischaemic heart disease.11,12 The benefit of CRT was seen mainly in patients with LBBB and a QRS duration >150 ms. The number needed to treat (NNT) with CRT to gain one life year over a period of three years is <7 and the NNT with CRT to reduce one hospitalisation is <4.13
CRT can be considered for patients with LVEF greater than 35% and symptoms of HF who have another indication for pacing.7 The BLOCK-HF (Biventricular versus Right Ventricular Pacing in Heart Failure Patients with Atrioventricular Block) study randomised patients who had high-degree AV block to receive either CRT or conventional RV pacing (n=691; NYHA I or II; LVEF, 35–50%). The primary end point of all-cause mortality, urgent HF visit or increase in LV end systolic volume index occurred in 56% of patients who received conventional RV pacing, compared to 46% of patients treated with a CRT device, demonstrating superiority of CRT over conventional RV pacing.14
There are several other groups of patients for whom CRT may be useful, but the evidence is less certain.
Does CRT work in moderate-to-severely symptomatic patients (NYHA class III/IV)?
CRT may be a useful treatment for patients with advanced HF; one study published from a single centre found that CRT device implantation in patients with severe LVSD and broad QRS complexes who are dependent on intravenous (IV) inotropic support can enable LV recovery to the point that patients are weaned off support and survive to discharge.15 It is important to note that guidelines recommend against the use of implantable defibrillators in patients with NYHA class IV symptoms.3,7
Does CRT work in patients with atrial fibrillation?
There is no evidence from RCTs that patients with atrial fibrillation (AF) benefit from CRT device implantation. The only trial recruiting a significant number of patients with AF – RAFT (Resynchronisation–Defibrillation for Ambulatory Heart Failure Trial) – showed no benefit in the AF subgroup.12
The subsequent CERTIFY study (Cardiac Resynchronisation Therapy in Atrial Fibrillation Patients Multinational Registry) compared patients in permanent AF undergoing CRT combined with either AV nodal ablation or rate-limiting medications to patients in sinus rhythm undergoing CRT (AF and AV node ablation [n=443]; AF and rate-limiting medication [n=895]; sinus rhythm [n=6,046]).16 Patients with AF and CRT who had undergone AV node ablation had a similar mortality rate to patients with CRT in sinus rhythm and lower mortality than patients with AF and CRT who were taking rate-limiting medications.16
Only one patient in RAFT had undergone AV junction ablation and it is possible that the neutral results were due to insufficient biventricular pacing in patients with AF.12
For every 1% increase in biventricular pacing percentage, the risk of mortality decreases by 6–10% among patients in sinus rhythm with CRT-D.17 Biventricular pacing percentage was found to be inversely associated with mortality in patients, regardless of underlying rhythm, in a large cohort study (n=36,935).18
Case series of patients with AF and LBBB suggests that CRT might be beneficial, particularly when strategies are used to maximise biventricular pacing rates, either pharmacologically or with AV node ablation.19
However, case series (whether collected together into a ‘meta-analysis’ or not) are no substitutes for clinical trials. Proper RCTs of different strategies for patients with LVSD, AF and LBBB are needed to prevent patients from being exposed to anecdote-based medicine.
Does CRT work in patients with narrow QRS complexes?
The Echo-CRT (Echocardiography Guided Cardiac Resynchronization Therapy) study (n=1,679) recruited patients with narrow QRS complexes and echocardiographic evidence of mechanical dyssynchrony.20 CRT did not confer any benefit to patients with narrow QRS complexes and was stopped early for futility.20 However, the trial was not included in the NICE Assessment Group’s systematic review, hence the inclusion of patients with QRS >120 ms (rather than >130 ms as in ESC guidance) in the NICE recommendations for CRT.3
Two individual patient data meta-analyses from the landmark trials of CRT have suggested the prognostic benefit of CRT was less certain with a QRS duration 130–149 ms, with a signal towards potential harm in patients with a QRS <130 ms (table 1).21,22
Table 1. Estimated hazard ratios for mortality, and mortality or HF hospitalisation based on QRS duration derived from an individual patient data meta-analysis in the landmark CRT clinical trials23
QRS duration (ms) | All-cause mortality HR (95% bootstrap CI) |
All-cause mortality or HF hospitalisation HR (95% bootstrap CI) |
<120 | 1.20 (0.90–1.70) | 1.50 (0.90–1.80) |
120–139 | 1.00 (0.90–1.40) | 1.10 (0.90–1.50) |
140–159 | 0.90 (0.80–1.10) | 0.90 (0.80–1.10) |
>160 | 0.80 (0.70–0.90) | 0.70 (0.60–0.80) |
Key: CI = confidence interval; CRT = cardiac resynchronisation therapy; HF = heart failure; HR = hazard ratio |
As a result, the most recent ESC HF guidelines have downgraded the recommendation for CRT in patients with LBBB and QRS duration 130–149 ms from class I (‘recommended’) to class IIa (‘should be considered’).7
Interestingly, this change was made between the 2016 and 2021 iterations of the guidelines, despite the absence of new data. Evidence, perhaps, of the problems implementing evidence from subgroup analyses into practice – can we base practice on subgroup findings within a trial or should we focus on patients who meet the trial eligibility criteria only? Subgroup analyses may be statistically underpowered. However, ignoring the potential benefits seen in subgroups may mean that patients who could benefit from a treatment miss out. The change in the guidelines recognises the weaknesses of subgroup analyses while acknowledging that there may be some patients who might benefit from a treatment, even though they do not necessarily meet trial eligibility criteria.
Does CRT work in patients with mild or moderate LVSD?
As with assessing efficacy of a new pharmacological intervention, such as an angiotensin-converting-enzyme inhibitor, initial trials concentrated on selective high-risk and/or highly symptomatic patient groups. This is also true of CRT. To date, no RCT has been published on the efficacy of CRT in patients with HF with a preserved ejection fraction, or mild or moderate LVSD. Studies are currently ongoing.24
Does CRT work in patients without LBBB?
An individual patient meta-analysis of all randomised controlled data of CRT (n=3,872) found that the reduction in mortality or hospitalisation with HF with CRT compared to medical treatment alone was only statistically significant in those with LBBB morphology (HR 0.66; 95% CI, 0.55–0.78) but not in patients with right bundle branch block (RBBB) (HR 0.74; 95% CI, 0.44–1.23) or non-specific interventricular conduction delay (HR 0.82; 95% CI, 0.48–1.41). However, the same analysis found that patients with longer QRS durations derived greatest benefit from CRT device implantation, regardless of QRS morphology. Additionally, there was a signal that CRT may be associated with greater risk of mortality or hospitalisation with HF in patients with QRS duration <130 ms.
The ESC and NICE subsequently attempted to distil these findings into usable clinical guidelines; the ESC guidelines recommend CRT for symptomatic patients with LBBB and QRS ≥130 ms (class I recommendation – ‘indicated’), but only advise consideration of CRT in patients with RBBB and QRS ≥130 ms (class IIb recommendation – ‘may be considered’). CRT is contraindicated in patients with QRS <130 ms.7 NICE recommend CRT for symptomatic patients with LBBB and QRS >120 ms with CRT only indicated in patients with RBBB if they have a QRS >120 ms and NYHA IV symptoms or QRS >150 ms.3
ICDs
Patients with HF are at increased risk of life-threatening ventricular arrhythmias.25 Amiodarone may reduce the incidence of ventricular arrhythmia but has no impact on overall mortality and is often associated with a high rate of toxicity, which limits its long-term use.26 Other anti-arrhythmic medications such as flecainide, dronedarone and disopyramide are contraindicated in patients with HF.27
ICDs reduce mortality in patients with HF and history of symptomatic, sustained ventricular arrhythmias.23,28 Prophylactic ICD implantation also reduces sudden cardiac death29 and mortality in patients with HFrEF due to ischaemic and non-ischaemic cardiomyopathy.30,31
How do ICDs work?32
ICDs consist of a ventricular lead positioned in the RV apex or septum and the generator. The ventricular lead can act as a standard pacing lead, but it also contains a shock coil. To deliver a shock, the device generates and discharges a large current across the heart from the tip of the ventricular lead to the generator. In some patients, a lead is also positioned in the right atrium if atrial pacing is required.
The ICD has two modes of action on ventricular arrhythmias – anti-tachycardia pacing (ATP) and shock. In the event that a patient with an ICD goes into ventricular tachycardia (VT), the device will attempt to ‘pace’ the heart out of the abnormal rhythm; the ventricular lead paces the RV at a rate greater than that of the ventricular rate of the arrhythmia. Programming within the generator then reduces the paced rate to that of a normal heart for a period of seconds thus terminating the arrhythmia. In the case of recurrent VT not amenable to ATP or VF, a shock is delivered by the ICD from the RV lead tip to the generator. Patients have variable awareness of ATP but will always know when the ICD has shocked them.
Patient selection and guidelines
ICDs are implanted for one of two reasons:
- a patient who has survived an episode of ventricular arrhythmia, which caused haemodynamic instability – both ESC and NICE guidelines recommend all such patients should be considered for a secondary prevention ICD;3,7
- patients with HF in whom sudden cardiac death is considered a high risk – making this decision is a lot more complex and requires careful patient selection.
Primary prevention ICD
ESC guidelines currently recommend ICD implantation for primary prevention in patients with symptomatic HFrEF (NYHA II and III) and LVEF ≤35% despite more than three months of optimal medical therapy.7 NICE recommends primary prevention ICD implantation in asymptomatic patients with LVEF ≤35% and QRS >120 ms.3 |
Survival benefit is uncertain for patients with LVEF ≥35% and in patients with mild HF (NYHA II), the NNT is high (around 50 devices are required to prevent one death per year).33 The intent of ICD implantation is to improve survival and thus the decision to treat should be taken in the context of the patient’s prognosis and their own view of their QoL.
In patients at high risk of sudden cardiac death, an ICD should be considered regardless of QRS duration.2,4 Both guidelines recommend careful evaluation of prognosis before ICD implantation.3,7
In patients with moderate or severe HF, a reduction in sudden death from prophylactic ICD implantation may be offset by an increase in death due to worsening HF.34 For example, ICD implantation is not recommended for patients with NYHA IV symptoms or those who are expected to die of other causes within a year.3,4,7
Complications and considerations
All patients should be counselled regarding procedural complications, inappropriate shocks, implications for driving and planning deactivation of the device.7
Box 2. Complications of ICD implantation:37,38
Complication | Incidence |
Lead displacement | 3.1% |
Generator-related complications | 2.7% |
Infection | 1.5% |
Haematoma | 1.2% |
Pneumothorax | 1.1% |
Inappropriate shocks | 5.0% |
Key: ICD = implantable cardioverter-defibrillator |
Inappropriate shocks used to be a common complication of ICDs (up to 40%), usually triggered by AF, other supraventricular tachycardias or artefact.35 Rates are far lower (~5%) with modern programming approaches.36
ICDs should be deactivated in patients with deteriorating HF, terminal illness or those with a ‘do not attempt cardiopulmonary resuscitation (DNACPR)’ order in place. Generator changes should only be undertaken after careful reassessment of treatment aims.4,7
Once a common cause of mortality amongst patients with HF, the rate of sudden cardiac death has fallen dramatically in recent years (figure 7).34 Advances in the treatment of risk factors for HF mean that patients are diagnosed at a much later stage in life and advances in the treatment of HF mean that, once diagnosed, patients live longer with the disease. As a result, there are many more competing causes of morbidity and mortality for patients with HF. Although ICDs remain in guidelines, the additional prognostic benefit alongside ‘quadruple therapy’ is unknown.
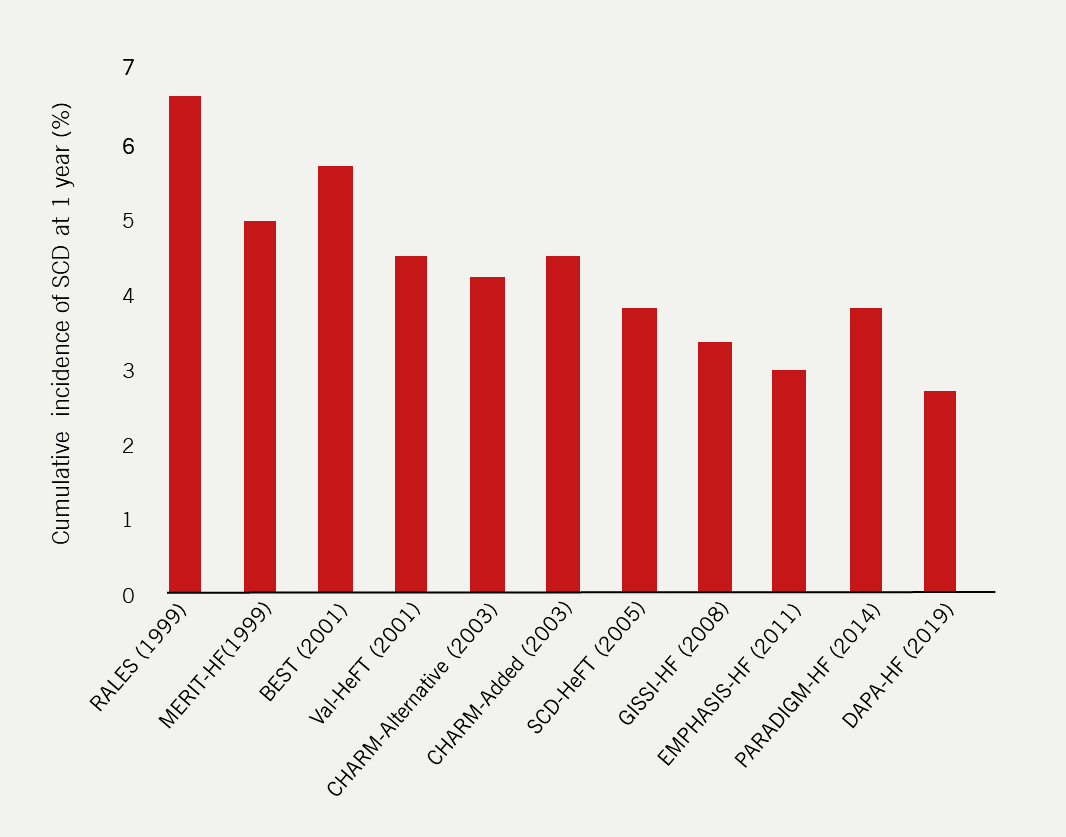
Data obtained from Shen et al., 201734 and McMurray et al., 201939 Key: BEST = Beta-Blocker Evaluation of Survival Trial; CHARM = Candesartan in Heart Failure – Assessment of Reduction in Mortality and Morbidity; DAPA-HF = Dapagliflozin in Patients with Heart Failure and Reduced Ejection Fraction; EMPHASIS-HF = Eplerenone in Mild Patients Hospitalization and Survival Study in Heart Failure; GISSI-HF = Gruppo Italiano per lo Studio della Sopravvivenza nella Insufficienza Cardiaca‐Heart Failure; MERIT-HF = Metoprolol CR/XL Randomised Intervention Trial in Congestive Heart Failure; PARADIGM-HF = Prospective comparison of ARNI with an Angiotensin-Converting Enzyme Inhibitor to Determine Impact on Global Mortality and Morbidity in Heart Failure; RALES = Randomized Aldactone Evaluation Study; SCD = sudden cardiac death; SCD-HeFT =-Sudden Cardiac Death in Heart Failure Trial; Val-HeFT = Valsartan Heart Failure Trial |
Driver and Vehicle Licencing Agency rules on ICDs and driving40
All patients with an ICD should be counselled on the impact on their fitness to drive and prescribers should consult the latest guidelines from the DVLA on ICDs and driving. Updated guidance available at: https://www.gov.uk/guidance/cardiovascular-disorders-assessing-fitness-to-drive#implantable-cardioverter-defibrillator-icd
Please note that by clicking this link, you will be taken to a third-party website.
Other device-based treatments
Multiple other device-based treatments have reached advanced stages of clinical trials, but very few have filtered through to clinical practice. This is perhaps a reflection of the difficulties in performing clinical trials of novel device-based treatments which require strict regulation and, often, sham procedures in the control arm to be statistically robust. It may also be that compared to modern medical therapies, which are comparatively cheap with no procedural risk and of large prognostic and symptomatic benefit, the expensive and risky implantation of newer device-based therapies cannot justify the small, incremental gains that they offer.
Invasive intra-cardiac pressure monitoring
The majority of patients admitted with HF have fluid retention – a consequence of increased intra-cardiac and pulmonary pressures, which may be detectable on invasive monitoring several weeks before overt congestion manifests. Several implantable devices have been developed that remotely measure intra-cardiac pressures.
Evidence
CardioMEMS™ device
The CHAMPION trial (CardioMEMS™ Heart Sensor Allows Monitoring of Pressure to Improve Outcomes in NYHA Class III Heart Failure Patients) (2011) of the CardioMEMS™ device (which measures pulmonary artery pressure) is the largest RCT of such devices to date.41 The investigators enrolled 550 patients (average age, 61 years; NYHA class III with an admission to hospital with HF within the preceding 12 months; ~20% of whom had an LVEF ≥40%) all of whom had the CardioMEMS™ device implanted.41 Patients were then assigned to either a ‘treatment’ group (in which investigators were aware of the pulmonary artery pressure) or a control group (in which investigators were ‘masked’ to the pulmonary artery pressures).41
HF medications and diuretics were titrated based on pulmonary artery pressure (where known) and patient symptoms and signs assessed over the telephone at pre-determined intervals.41 There was a 28% reduction in the primary end point of the rate HF hospitalisation after six months in the treatment group compared to the control group.41
Longer-term outcomes were similarly better in the treatment group compared to the control group with reductions in HF hospitalisation rate (HR 0.67; 95% CI, 0.55–0.80; p<0.0001), all-cause hospitalisation rate (HR 0.84; 95% CI, 0.75–0.95; p=0.0003), and combined end point of death or HF hospitalisation rate (HR 0.77; 95% CI, 0.60–0.98; p=0.03) during the 18-month randomised-access period.42 However, there was no mortality benefit with continuous pulmonary artery monitoring (19% vs. 23%; p=0.23).42
The GUIDE-HF trial (Haemodynamic-Guided Management of Heart Failure) (2021) of the CardioMEMS™ device enrolled 1,000 patients (average age, 70 years; 94% NYHA class II/III; median LVEF, 38%; 45% of whom had an LVEF >40%; median NT-proBNP, 1,480 ng/L).43 All patients had the device implanted and were then randomised to standard of care or care guided by haemodynamic monitoring from the device.43 The primary end point was total HF events (hospitalisation plus urgent care visits) or all-cause mortality measured at 12 months. There was no difference in the primary end point after 12 months.43
However, the authors reported a ‘COVID-19 (coronavirus disease 2019) sensitivity analysis’ in which they excluded all events that occurred after the onset of the COVID-19 pandemic.43 The authors reported a 19% reduction in the primary end point, driven entirely by a reduction in HF hospitalisations with no effect on either urgent hospital visits for HF, or death.43 The finding is counterintuitive and cannot be used to justify the use of the device beyond the findings of the whole trial.43 The COVID-19 pandemic led to national lockdowns and a reduction in patients having contact with healthcare providers. The CardioMEMS™ device, which allows for remote detection of early decompensation and titration of medications, is perfect for such a situation. However, all benefit from the device was observed in the weeks and months before lockdowns came into effect. The device had no impact on outcomes during the time it was designed to have the greatest impact.
It remains unknown whether the costs involved in implanting, monitoring and maintaining the devices are outweighed by the clinical benefit.
The MultiSENSE trial (Multisensor Chronic Evaluation in Ambulatory Heart Failure Patients) (n=900; average age, 66 years; 94% NYHA II–III; median NT-proBNP, 754 ng/L) found that an algorithm using variables measured by pre-implanted CRT-D devices could identify patients at greater risk of HF-related events (death, hospitalisation with HF, increased diuretic treatment without hospitalisation).44 Remote identification of patients at greater risk may allow earlier intervention that reduces the rate of adverse outcomes, although this was not tested in the study.44
HeartPOD™ device
The HOMEOSTASIS trial (Hemodynamically Guided Home Self-Therapy in Severe Heart Failure Patients) (n=45; average age, 66 years; average LVEF, 32%; NYHA II–III) established the safety and potential outcome benefits of titration of HF medications based on readings from an implantable pressure monitor in the left atrium (HeartPOD™).45 Medication changes depending on readings had been pre-programmed into the device by investigators with the broad aim of reducing or withdrawing diuretics from patients with low left atrial pressures or increasing diuretic in those with high pressures.45 These changes could be implemented by patients based on instructions from the device without involvement of the medical team.45
The LAPTOP-HF study (Left Atrial Pressure Monitoring to Optimize Heart Failure Therapy Study) of the HeartPOD™ device in patients with HFrEF and NYHA class III symptoms was stopped early due to a high rate of procedure-related complications upon device insertion.46 Amongst the 486 patients enrolled (out of a planned 730) titration of treatment based on left atrial pressure was associated with a 41% reduction in the risk of admission with HF compared to the control group (p=0.005).46
One problem with the above trials, and indeed with home telemonitoring in general, is that each device produces a large volume of data, much of which is difficult to interpret remotely from the clinical context. Another issue is that of the time taken to review and act upon the data generated; it is quite possible that by the time a doctor or nurse has had a chance to review the data and make a clinical decision, the adverse event predicted (e.g. hospitalisation with HF) has already occurred.
Patient-directed titration of HF medication (such as in HOMEOSTASIS and LAPTOP-HF) would ultimately bypass this step and may prove beneficial in the future. Interest in remote monitoring remains high and the advent of ‘wearable technology’ may lead to devices that can direct patients to manage their condition without the time-consuming involvement of their overstretched medical team or the risks associated with implantable devices.
Cardiac contractility modulation
A cardiac contractility modulation (CCM) device is an implantable device that delivers a low-voltage, biphasic current to the myocardium after the detection of the QRS complex during the ventricular refractory period.47 The result is to increase the strength of LV contraction without triggering a new action potential. Implantation is similar to that of a simple pacemaker with right atrial and ventricular leads.
Early studies suggest that CCM devices can improve exercise tolerance and QoL in patients with HFrEF, NYHA class II–IV symptoms and narrow QRS complex (<120 ms). However, despite being available for over a decade, the development in the technology has progressed slowly and there is no evidence of benefit on mortality or morbidity.48 More work is needed.
Neuromodulation
Recent years have seen a variety of implantable devices developed that are able to increase vagal tone by electrical stimulation of different nerve fibres. Increased vagal tone (activation of the parasympathetic nervous system) may be potentially beneficial for patients with HF in that it may counteract some of the deleterious effects of sympathetic activation.
Evidence
Four different methods of neuromodulation have been tested in patients with HF: spinal cord stimulation, carotid sinus nerve stimulation or baroreflex activation therapy, cervical vagal nerve stimulation and intracardiac AV-nodal stimulation. There are few trials of note:
- The DEFEAT-HF trial (Diagnosis and Determining the Etiology, Fluid Volume Must Be Assessed to Achieve Euvolemia, and Ejection Fraction Must Be Determined to Guide Therapy) of implantable spinal cord stimulators (n=66; average age, 61 years; average LVEF, 27%) found no change in biomarkers, echocardiographic or exercise variables, functional capacity or QoL with spinal cord stimulation compared to medical treatment.49
- Conversely, the Barostim HOPE4HF study (Hope for Heart Failure) (n=146; average age, 64 years; average LVEF, 24%; median NT-proBNP, 1,422 ng/L) found that an implantable device that stimulated the baroreceptors in the carotid sinus – thus reducing sympathetic tone and increasing parasympathetic activity – was associated with improved exercise capacity, QoL, symptom severity and NT-proBNP levels six months after implantation compared to medical therapy alone.50
- The INOVATE-HF study (Increase of Vagal Tone in Heart Failure) of a similar device in patients with HFrEF (n=707; average age, 61 years; average LVEF, 24%) demonstrated improvements in QoL, symptom severity and exercise capacity.51 However, there was no effect on the primary end point of mortality or HF hospitalisation rate, which, alongside a trend towards harm in the treatment arm, led to early termination of the study.51
More work will be required to clarify what, if any, role neuromodulation may have for patients with HF. While there are many devices available the failure and early termination of the largest study to date (INOVATE-HF) may signal the beginning of the end for neuromodulation as a treatment for HF.52
Treatment of sleep-disordered breathing in HF
Sleep disordered breathing (SDB) is a description of abnormal breathing patterns during sleep, affecting up to half of patients with HF. The condition is associated with a poor prognosis.53
There are two major types of SDB:
- obstructive (upper airway collapses but respiratory effort continues)
- central (respiratory drive ceases).
Evidence
Trials investigating the treatment of SDB with adaptive servo-ventilation in patients with acute (CAT-HF [Cardiovascular Outcomes with Minute Ventilation-Targeted Adaptive Servo-Ventilation Therapy in Heart Failure]) and chronic HF (SERVE-HF [Treatment of Predominant Central Sleep Apnoea by Adaptive Servo Ventilation in Patients with Heart Failure]) have failed to show outcome benefit.54,55 In fact, treatment with adaptive servo-ventilation was associated with increased risk of all-cause and cardiovascular mortality in SERVE-HF.55
The reason for the increased mortality in SERVE-HF is not clear and appeared to be related to an increase in the risk of sudden death in the treated patients.55 Adaptive servo-ventilation should certainly not be used routinely for patients with central sleep apnoea, although continuous positive airways support for patients with symptomatic obstructive sleep apnoea should be considered on an individual basis.
Ultrafiltration
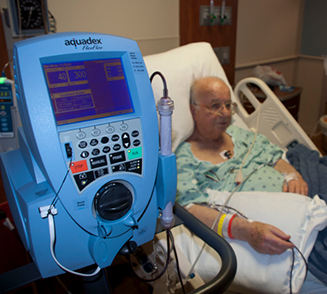
With kind permission from Mercy Health, Chesterfield, Missouri, USA |
Ultrafiltration as a treatment of venous congestion in HF was first investigated in the 1970s.56 It has since been the focus of RCTs to explore its possible role as an adjunct or even potential alternative to loop diuretics in patients with severe fluid retention (figure 8).
What is ultrafiltration?56
Ultrafiltration is an extracorporeal process that attempts to mimic filtration in the Bowman’s capsule. Venous blood is removed from the body and passed through a circuit with a membrane permeable to smaller molecules, such as water and electrolytes. Blood is maintained at a pressure greater than the transmembrane pressure; hydrostatic pressure forces water and solutes to cross the membrane and out of the blood. The intravascular space is then refilled with fluid from the interstitium, thus reducing congestion.
It differs from dialysis in that the latter is usually intermittent, allowing solutes – which can be larger-sized molecules (e.g. toxins, lactate) – to diffuse through a semi-permeable membrane down the solute’s concentration gradient into the dialysate fluid.
By using ultrafiltration, fluids can be removed rapidly – at rates of up to 500 ml/hour (up to 9.6 L/day) – although, in practice, 150–300 ml/hour (3.6–7.2 L/day) is considered adequate. Lower rates are advised when there is RV disease or pulmonary arterial hypertension as there is a higher risk for potential haemodynamic disturbance with high fluid-removal rates.
Ultrafiltration rate should be monitored and adjusted according to clinical variables such as blood pressure. Serial haematocrit measurements can help monitor adequate refilling of the intravascular space with fluid from the interstitium.57,58
By ensuring that intravascular volume does not decrease (i.e. ultrafiltration rate does not exceed plasma refilling), further renin-angiotensin-aldosterone-system activation, hypotension and renal injury may be prevented.59 Another advantage of ultrafiltration is that more sodium can be removed (and less potassium) compared to diuretic therapy.60
With newer machines, ultrafiltration can be performed using peripheral or central venous access. The typical treatment period may vary from 24–72 hours, mainly limited by the fact that the ultrafiltration membranes only last up to 72 hours. Diuretics are stopped during ultrafiltration. Urine output can be monitored but it is not essential to do so.
Evidence
To date, there are three key trials of ultrafiltration in patients with severe fluid retention: UNLOAD (Ultrafiltration Versus Intravenous Diuretics for Patients Hospitalised for Acutely Decompensated Heart Failure), CARRESS-HF (Effectiveness of Ultrafiltration in Treating People with Acute Decompensated Heart Failure and Cardiorenal Syndrome) and AVOID-HF (Aquapheresis Versus Intravenous Diuretics and Hospitalizations for Heart Failure) studies.61–63
In the UNLOAD trial, investigators enrolled 188 patients admitted with HF (average age, 62 years; median BNP 1,256 ng/L in the ultrafiltration arm).61 Patients were randomised to either ultrafiltration or loop diuretic therapy within 24 hours of hospitalisation.61 The co-primary end points were weight loss and dyspnoea relief at 48 hours with net fluid loss and rehospitalisation with HF after 90 days among the pre-specified secondary end points.61 The ultrafiltration group had greater weight and fluid loss (5.0 kg vs. 3.1 kg, p<0.001 and 4.6 L vs. 3.3 L, p=0.001 respectively), but there was no difference in patient-reported dyspnoea.61 Rehospitalisation rate was lower in the ultrafiltration arm, but the trial was not powered to detect a significant difference. There was significantly less hypokalaemia and also no difference in serum creatinine with ultrafiltration compared with diuretic treatment.61
In the CARRESS-HF trial, investigators enrolled 188 patients admitted with HF and deterioration in renal function from baseline (median age, 69 years; median LVEF, 30%; median NT-proBNP, 5,013 ng/L in the ultrafiltration arm).62 Patients were randomised to titration of IV diuretic treatments (plus treatment with a thiazide diuretic, vasopressors or inotropes) based on signs and symptoms, or ultrafiltration at a rate of 200 ml/hr.62 Treatment was continued until the signs and symptoms of congestion were reduced at the judgement of the treating doctor. The co-primary end point was change in serum creatinine and change in weight at 96 hours.62 Both groups lost a similar amount of weight, but serum creatinine was significantly higher in the ultrafiltration treatment group compared to the pharmacological treatment group (20.3 µmol/L vs. −3.5 µmol/L; p=0.003).62
It is difficult to know what the correct interpretation of the CARRESS-HF trial is. Factors suggesting that ultrafiltration might not be harmful (as is commonly interpreted) include:
- there was substantial dropout and cross-over of patients from the ultrafiltration to the pharmacological treatment arm in the first 24 hours62
- although 90% of patients enrolled in the trial had residual congestion at the time of evaluation of the primary end point (96 hours), only 32% of patients in the ultrafiltration arm were still receiving their assigned treatment compared to 80% of patients in the pharmacological arm62
- a recent per-protocol analysis of the CARRESS-HF data found that ultrafiltration was associated with greater cumulative (p=0.003) and net (p=0.001) fluid loss, and greater weight loss relative to baseline (p=0.02) compared to pharmacological therapy62
- rather than being an indicator of disease progression or harmful treatment, a transient increase in serum creatinine during decongestion may actually indicate more effective fluid loss and is associated with better post-discharge outcomes than in patients with no change or a decrease in serum creatinine levels.64
In the AVOID-HF trial, investigators aimed to enrol ~800 patients admitted with HF who would be randomised to ultrafiltration or conventional therapy. Unfortunately, the trial was terminated early by the sponsor with only 224 patients enrolled, so although the primary end point of HF event at 90 days favoured ultrafiltration, the effect was not statistically significant.63
The role of ultrafiltration in HF remains uncertain and further research is required. Ultrafiltration is expensive in terms of equipment and staff training and the economic impact of the therapy as an initial strategy for acute HF may limit its use.65 However, early ultrafiltration might reduce length of stay for acute HF, which currently stands at 8–9 days in the UK. Halving length of stay would make ultrafiltration appear a very attractive alternative to conventional treatment.
The ESC guidelines recommend that ultrafiltration may be used in patients who fail to respond to diuretics or have end-stage renal failure and its complications.7
Box 3. Clinical scenarios in which ultrafiltration may be indicated
Venous congestion resistant to high-dose diuretic therapy |
Oliguria refractory to fluid resuscitation |
Refractory severe hyperkalaemia (>6.5 mmol/L) |
Acidaemia (pH <7.2) |
Renal failure |
Urea >25 mmol/L |
Creatinine >300 μmol/L |
Box 4. Patients in whom ultrafiltration is contraindicated56
Septicaemia |
Poor venous access |
Cardiogenic shock requiring inotropic support |
Advanced renal disease (consider renal replacement therapy instead, such as haemofiltration or peritoneal dialysis) |
Box 5. Potential complications of treatment with ultrafiltration56
Venous line infection |
Sepsis |
Increased risk of haemorrhage as patients are anticoagulated with heparin |
Haemodynamic disturbance with hypotension |
Acute kidney injury |
Future treatment pipeline
Gene and stem cell therapy
On a cellular level, chronic HF is associated with activation of stress-signalling pathways which alter gene expression in the failing cardiomyocytes through changes in transcription factors, co-regulators and microRNAs. These changes contribute to the loss of functioning myocytes.66
Gene and stem cell therapy for HF is in its infancy, but much laboratory research supports its possible role. There are many possible genetic targets and gene therapy may become a useful treatment in HF. Similarly, stem cell therapy to replace dead myocardium may yet find a role in HF management. However, early clinical trials have been disappointing and there are still problems to overcome.
Gene therapy
The underlying trigger for the subsequent changes in chronic HF caused by the stress signalling pathway is, in part, chronic stimulation of beta-adrenergic receptors (β-ARs) leading to alterations in gene expression by cyclic adenosine monophosphate (cAMP)-dependent signalling pathways.66
Some of the changes in gene expression are currently being exploited as biomarkers in HF: for example, up-regulation of the human natriuretic peptide B (NPPB) gene for NT-proBNP. However, many of the changes to gene expression in HF affect functional proteins and are damaging to the heart.
Gene therapy is ‘the introduction or alteration of genetic material within a cell or organism with the intention of curing or treating a disease’,65,67 and may allow direct modification of the damaging changes to gene expression that are associated with HF.
In therapeutic terms, this either increases the production of a deficient protein − commonly by delivering a new copy of the relevant gene − or inhibits production of a deleterious protein, for example by interfering with RNA.
Although there is much enthusiasm for gene therapy and much promising laboratory data, there are no convincing clinical trial data in humans as yet.
Potential targets for gene therapy66
A plethora of changes occur in gene expression in HF affecting proteins involved in the following:
- contractile proteins (α- and β-myosin heavy chains)
- cytoskeleton (titin and tubulin)
- pro-inflammatory cytokines (tumour necrosis factor alpha [TNF-ɑ] and interleukin [IL]-6)
- receptors (β-ARs and ryanodine receptors)
- transporters (sarcoendoplasmic reticulum calcium adenosine triphosphatase [SERCA] and Na+/K+ -adenosine triphosphatase)
- metabolism switches from free fatty acids to carbohydrate (glucose transporter [GLUT], pyruvate dehydrogenase kinase [PDK])
- ion channels (Nav1.5 and Kir)
- calcium binding proteins (calmodulin and phospholamban)
- exchangers (sodium-calcium exchanger [NCX] and sodium/proton exchangers [NHX])
- kinases (CaMKII).
Vectors and delivery systems for gene therapy68
Once the therapeutic gene has been selected, an appropriate vector and delivery method is required. There are various techniques used in laboratory research (table 2) but in the small numbers of clinical trials that have been carried out, the most common vector is the adeno-associated virus and the delivery method is the antegrade intracoronary infusion without balloon occlusion.
Table 2. The variety of vectors and delivery methods available to deliver genetic material
Advantages | Disadvantages | |
Vectors | ||
Non-viral | Low cost, non-pathogenic and non-immunogenic | Low transfection efficiency and lack of cardiotropism |
Adenovirus | Easy to produce and all major cardiac cell types efficiently transduced | Immune and inflammatory reactions, short-term gene expression and a tendency to infect all organs |
Adeno-associated virus | Non-pathogenic, minimal immunogenicity long term gene expression and cardiac tropism | Presence of neutralising antibodies limits the suitability of this vector to approximately 50% of heart failure patients |
Retrovirus | Long-term gene expression | Inability to transduce non-dividing cells such as cardiac myocytes |
Delivery methods | ||
Antegrade intracoronary infusion without coronary artery occlusion | Homogenous delivery to whole myocardium. Tolerated in patients with heart failure |
Limited in patients with significant coronary artery disease |
Antegrade intracoronary infusion with coronary artery occlusion | Homogenous delivery to whole myocardium. Allows flow of vector to occur without dilution |
Limited in patients with significant coronary artery disease |
Circulatory perfusion technique | Vector has a longer exposure time to myocardium | Complicated system and requires anticoagulation |
Retrograde infusion through coronary sinus | High transduction efficiency | High coronary pressure can result in myocardial oedema or haemorrhage |
Direct myocardial injection | Avoids first pass of liver, the effect of neutralising antibodies and the inflammatory response | Limited vector delivery due to restricted area of injection |
Peripheral intravenous infusion | Simplest, most convenient delivery method | Dilutional effect and no vector with high enough cardiotropism to be clinically viable currently |
Clinical experience of gene therapy
Numerous animal studies have targeted various genes and used a variety of vectors and delivery methods. The genes which have been successfully targeted encode for proteins involved predominantly in either calcium-handling (sarcoplasmic [endoplasmic] reticulum calcium adenosine triphosphatase (SERCA2a), phospholamban (PLB), protein phosphatase 1, inhibitor-1, S100A1 and small ubiquitin-related modifier 1) or the β-adrenergic system (upregulation of β-ARs, inhibition of G-protein-coupled receptor kinases, activation of adenyl cyclase).68
Many of these laboratory studies have shown promise, but the only area which has advanced to clinical trials targets the SERCA2a gene using an adeno-associated virus as the vector, delivered during an intracoronary infusion. The function of SERCA2a in the normal physiology versus HF state.69
Typical cycling of Ca2+ within cardiac cells commences with the depolarization of the cardiac action potential, leading to a minor influx of Ca2+ into the cytoplasm via L-type Ca2+ channels.69 Subsequently, this initiates a larger release of Ca2+ from the sarcoplasmic reticulum (SR) reservoir through the calcium release channel (CaRC), with Calstabin (FKB12.6) playing a role in stabilising CaRC function.69 This calcium-induced release further stimulates contraction by binding with troponin C in the cardiac myofilaments. During relaxation (diastole), Ca2+ is reabsorbed into SERCA2a and expelled from the cell via the NCX, with the function of SERCA2a regulated by PLB.69
In HF, abnormalities in Ca2+ regulation occur, including diminished expression of SERCA2a and inhibition of its function by PLB, resulting in compromised Ca2+ reuptake during diastole, heightened diastolic tension, and impaired relaxation, leading to reduced availability of Ca2+ for contraction and weakened contractions.69 Although PLB levels remain unchanged in HF, reduced phosphorylation of PLB intensifies its inhibition of SERCA2a, leading to an accumulation of cytosolic Ca2+, which is offset by an increase in NCX expression. Additionally, hyperphosphorylation of CaRCs by protein kinase A or CaMKII disrupts FKB12.6 association, destabilising CaRC and promoting Ca2+ leakage during diastole. This combination of increased Ca2+ extrusion via NCX and elevated leakage from CaRC during diastole fosters delayed after depolarisations and triggered activity, which may precipitate ventricular arrhythmias.69
However, results have been disappointing; phase II trials, CUPID 2 (Calcium Up-Regulation by Percutaneous Administration of Gene Therapy in Cardiac Disease Phase 2b) and AGENT-HF (AAV1-CMV-SERCA2a Gene Therapy Trial in Heart Failure), failed to show an improvement in ventricular dimensions or outcome with gene therapy that restored function of SERCA2a in patients with HFrEF.70,71
One frequently cited reason for the failure of both CUPID 2 and AGENT-HF was the relatively low myocardial uptake of the viral vectors compared to the pre-clinical animal studies.68 Advancements in more ‘cardiotropic’ vectors mean that work to find an effective genetic therapy for patients with HF continues.
Genetic therapies have been proposed as a potentially curative treatment for heritable causes of HF such as muscular dystrophy but translating promising results in animal models into clinical trials is proving problematic.72
Finally, there is ongoing interest in ‘gene-silencing’ therapies for transthyretin (ATTR) amyloidosis, an increasingly recognised cause of HF with normal ejection fraction in elderly patients. Production of transthyretin occurs in the liver and can be interrupted by RNAs specifically engineered to inhibit translation of the gene. Three such so-called ‘small interfering RNAs’ exist; revusiran, inotersen and patisiran.73
Treatment with inotersen or patisiran can improve various neurological end points in patients with hereditary ATTR and polyneuropathy compared to placebo.74,75 However, none of these studies had HF-related end points and enrolment into the only study involving patients with cardiac amyloidosis, the ENDEAVOUR study (Study to Evaluate the Efficacy and Safety of AZD4831 in Participants with Heart Failure with Left Ventricular Ejection Fraction >40%) of revusiran, was halted due to safety concerns arising from the phase II extension study in which more than half of patients receiving revusiran developed peripheral neuropathy.76
The APOLLO trial (Study of an Investigational Drug, Patisiran [ALN-TTR02], for the Treatment of Transthyretin (TTR)-Mediated Amyloidosis) of patisiran in patients with hereditary ATTR cardiac amyloidosis (n=126; mean age, 61; median NT-proBNP, 837 ng/L; 40% of whom had NYHA I symptoms) found a very small but statistically significant improvement in global longitudinal strain with patisiran over placebo after 18 months of treatment.77 It is unlikely that such a small change in one isolated marker of left ventricular function would lead to any clinical benefit. More work is needed.
Stem cell therapy
On the cellular level, HF is characterised by the loss of functioning myocytes. Regenerating myocardial tissue with cell therapies is a potential future treatment for HF. Types of cells under investigation include bone marrow mononuclear and mesenchymal stem cells, skeletal myoblasts and cardiac progenitor cells.66
The field is littered with many small studies conducted in single centres with no control groups; the promising preclinical data is yet to translate into convincing benefit in phase II and III trials. Insufficient retention of the cells (delivered by injection or infusion) is a significant problem and generation of ‘new’ myocardium is yet to be demonstrated.78,79 Ventricular arrhythmia is a common adverse event in clinical trials, and the trials have only involved a very select group of patients. The safety of stem cell therapy has not yet been confirmed. Additionally, ethical issues surrounding the use of human embryonic stem cells may limit further work in the field.
Summary
CRT with or without an ICD are useful treatment options for patients who are either on OMT or who are unable to tolerate optimal medical therapy. Implantation is associated with procedural risk, which must always be weighed against potential benefit of implantation, particularly regarding ICD implantation. Aside from the established device therapy, implantable pulmonary artery monitors may have a role in disease management, but this has not been clarified by trials to date; the technology is expensive and the benefit minimal. Other devices, such as left atrial pressure monitors and vagus nerve stimulators, are unlikely to be used in patients with HF. Similarly, despite its early promise, trials of gene therapy and stem cell therapy for patients with HF to date have been unremarkable and such treatments are a long way from being used in practice.
Table 3. Treatment summary of the invasive non-surgical treatment of heart failure based on the NICE guidelines
Device/therapy | Indications1,80 | Complications37,38,81 | |||||
NYHA class | LVEF ≤35% | ||||||
QRS duration | I | II | III | IV | Procedural complications e.g. infection, haematoma, lead complications, pneumothorax, haemothorax, brachial plexus injury Tricuspid regurgitation Failure to implant LV lead Coronary sinus dissection Cardiac perforation / tamponade Extracardiac / phrenic nerve stimulation LV lead dislodgement Deep vein thrombosis Death |
||
CRT with or without defibrillator (CRT-D) or pacing (CRT-P) | <120 ms | ||||||
120–149 ms, no LBBB |
CRT-P | ||||||
120–149 ms, LBBB present |
CRT-D | CRT-P/-D | CRT-P | ||||
≥150 ms, ± LBBB |
CRT-D | CRT-D | CRT-P/-D | CRT-P | |||
ICD | <120 ms | If there is a high risk of sudden cardiac death | Lead displacement Generator-related complications Infection Haematoma Pneumothorax Inappropriate shocks |
||||
120–149 ms, no LBBB |
ICD | ICD | ICD | ||||
120–149 ms, LBBB present |
ICD | ||||||
≥150 ms, ± LBBB |
|||||||
Ultrafiltration |
|
Venous line infection Sepsis Increased risk of haemorrhage due to anticoagulation with heparin Haemodynamic disturbance with hypotension Acute kidney injury |
|||||
Key: CRT = cardiac resynchronisation therapy; D = defibrillation; ICD = implantable cardioverter-defibrillator; LV = left ventricular; LVEF = left ventricular ejection fraction; NYHA = New York Heart Association; P = pacing |
close window and return to take test
References
- Clark AL, Goode K, Cleland JG. The prevalence and incidence of left bundle branch block in ambulant patients with chronic heart failure. Eur J Heart Fail 2008;10:696–702. https://doi.org/10.1016/j.ejheart.2008.05.001 [Epub ahead of print]
- Nikolaidou T, Pellicori P, Zhang J, Kazmi S, Goode KM, Cleland JG, Clark AL. Prevalence, predictors, and prognostic implications of PR interval prolongation in patients with heart failure. Clin Res Cardiol 2018;107:108–19. https://doi.org/10.1007/s00392-017-1162-6
- National Institute for Health and Care Excellence. Implantable cardioverter defibrillators and cardiac resynchronisation therapy for arrhythmias and heart failure. NICE technology appraisals [TA314]. London: NICE, 2014. Available from: http://guidance.nice.org.uk/TA314 (accessed 25 October 2023)
- Khidir MJ, Delgado V, Ajmone Marsan N, Schalij MJ, Bax JJ. QRS duration versus morphology and survival after cardiac resynchronization therapy. ESC Heart Fail 2017;4:23–30. https://doi.org/10.1002/ehf2.12122 [Epub ahead of print]
- Kanzaki H, Bazaz R, Schwartzman D, Dohi K, Sade LE, Gorcsan J. A mechanism for immediate reduction in mitral regurgitation after cardiac resynchronization therapy: Insights from mechanical activation strain mapping. J Am Coll Cardiol 2004;44:1619–25. https://doi.org/10.1016/j.jacc.2004.07.036
- Sohaib SM, Chen Z, Whinnett ZI et al. Meta-analysis of symptomatic response attributable to the pacing component of cardiac resynchronization therapy. Eur J Heart Fail 2013;15:1419–28. https://doi.org/10.1093/eurjhf/hft139
- McDonagh TA, Metra M, Adamo M et al; Task Force for the diagnosis and treatment of acute and chronic heart failure of the European Society of Cardiology. 2021 European Society of Cardiology Guidelines for the diagnosis and treatment of acute and chronic heart failure. (Correction published: Eur J Heart 2021;42:4901. https://doi.org/10.1093/eurheartj/ehab670) Eur J Heart 2021;42:3599–726. https://doi.org/10.1093/eurheartj/ehab368
- Pothineni NVK, Gondi S, Cherian T et al. Complications of cardiac resynchronization therapy: comparison of safety outcomes from real-world studies and clinical trials. J Innov Card Rhythm Manag 2022;13:5121–5. https://doi.org/10.19102/icrm.2022.13080
- Anand IS, Carson P, Galle E et al. cardiac resynchronization therapy reduces the risk of hospitalizations in patients with advanced heart failure results from the comparison of medical therapy, pacing and defibrillation in heart failure (COMPANION) Trial. Circulation 2009;119:969–77. https://doi.org/10.1161/CIRCULATIONAHA.108.793273 [Epub ahead of print]
- Cleland JG, Freemantle N, Erdmann E et al; CARE-HF Study Investigators. Long-term mortality with cardiac resynchronization therapy in the Cardiac Resynchronization-Heart Failure (CARE-HF) trial. Eur J Heart Fail 2012;14:628–34. https://doi.org/10.1093/eurjhf/hfs055 [Epub ahead of print]
- Moss AJ, Hall WJ, Cannom DS et al; MADIT-CRT Trial Investigators. Cardiac-resynchronization therapy for the prevention of heart-failure events. N Engl J Med 2009;361:1329–38. https://doi.org/10.1056/NEJMoa0906431 [Epub ahead of print]
- Tang ASL, Wells GA, Talajic M et al; RAFT Investigators. Cardiac-resynchronization therapy for mild-to-moderate heart failure (RAFT). N Engl J Med 2010;363:2385–95. https://doi.org/10.1056/NEJMoa1009540
- McAlister FA, Ezekowitz JA, Wiebe N et al. Systematic review: cardiac resynchronization in patients with symptomatic heart failure. Ann Intern Med 2004;141:381–90. https://doi.org/10.7326/0003-4819-141-5-200409070-00101 Erratum in: Ann Intern Med 2005;142:311.
- Curtis AB, Worley SJ, Adamson PB et al. Biventricular pacing for atrioventricular block and systolic dysfunction (BLOCK-HF). N Engl J Med 2013;368:1585–93. https://doi.org/10.1056/NEJMoa1210356
- Cowburn PJ, Patel H, Jolliffe RE, Wald RW, Parker JD. Cardiac resynchronization therapy: an option for inotrope-supported patients with end-stage heart failure? Eur J Heart Fail 2005;7:215–7. https://doi.org/10.1016/j.ejheart.2004.11.005
- Gasparini M, Leclercq C, Lunati M et al. Cardiac resynchronization therapy in patients with atrial fibrillation: the CERTIFY study (cardiac resynchronization therapy in atrial fibrillation patients multinational registry). JACC Heart Fail 2013;1:500–7. https://doi.org/10.1016/j.jchf.2013.06.003 [Epub ahead of print]
- Ruwald A-C, Kutyifa V, Ruwald MH et al. The association between biventricular pacing and cardiac resynchronization therapy-defibrillator efficacy when compared with implantable cardioverter defibrillator on outcomes and reverse remodelling. Eur Heart J 2015;36:440–8. https://doi.org/10.1093/eurheartj/ehu294 [Epub ahead of print]
- Hayes DL, Boehmer JP, Day JD et al. Cardiac resynchronization therapy and the relationship of percent biventricular pacing to symptoms and survival. Heart Rhythm 2011;8:1469–75. https://doi.org/10.1016/j.hrthm.2011.04.015 [Epub ahead of print]
- Ganesan AN, Brooks AG, Roberts-Thomson KC, Lau DH, Kalman JM, Sanders P. Role of AV nodal ablation in cardiac resynchronization in patients with coexistent atrial fibrillation and heart failure a systematic review. J Am Coll Cardiol 2012;59:719–26. https://doi.org/10.1016/j.jacc.2011.10.891
- Ruschitzka F, Abraham WT, Singh JP et al; EchoCRT Study Group. Cardiac-resynchronization therapy in heart failure with a narrow QRS complex. N Engl J Med 2013;369:1395–405. https://doi.org/10.1056/NEJMoa1306687 [Epub ahead of print]
- Cleland JG, Abraham WT, Linde C et al. An individual patient meta-analysis of five randomized trials assessing the effects of cardiac resynchronization therapy on morbidity and mortality in patients with symptomatic heart failure. Eur Heart J 2013;34:3547–56. https://doi.org/10.1093/eurheartj/eht290 [Epub ahead of print]
- Woods B, Hawkins N, Mealing S et al. Individual patient data network meta-analysis of mortality effects of implantable cardiac devices. Heart 2015;101:1800–6. https://doi.org/10.1136/heartjnl-2015-307634 [Epub head of print]
- Connolly SJ, Hallstrom AP, Cappato R et al. Meta-analysis of the implantable cardioverter defibrillator secondary prevention trials AVID, CASH and CIDS studies. Eur Heart J 2000;21:2071–8. https://doi.org/10.1053/euhj.2000.2476
- ClinicalTrials.gov. Cardiac resynchronisation therapy versus rate-responsive pacing in heart failure with preserved ejection fraction (PREFECTUS). 2023. Available at: https://clinicaltrials.gov/study/NCT03338374?term=NCT03338374&rank=1#study-overview (accessed 30 January 2024)
- Saxon LA, Bristow MR, Boehmer J et al. Predictors of sudden cardiac death and appropriate shock in the comparison of medical therapy, pacing, and defibrillation in heart failure (COMPANION) trial. Circulation 2006;114:2766–72. https://doi.org/10.1161/CIRCULATIONAHA.106.642892 [Epub ahead of print]
- Singh SN, Fletcher RD, Fisher SG et al. Amiodarone in patients with congestive heart failure and asymptomatic ventricular arrhythmia. Survival Trial of Antiarrhythmic Therapy in Congestive Heart Failure. N Engl J Med 1995;333:77–82. https://doi.org/10.1056/NEJM199507133330201
- Santangeli P, Rame JE, Birati EY, Marchlinski FE. Management of ventricular arrhythmias in patients with advanced heart failure. J Am Coll Cardiol 2017;69:1842–60. https://doi.org/10.1016/j.jacc.2017.01.047
- Wyse DG, Friedman PL, Epstein AE; the Anti-arrhythmics versus Implantable Defibrillators (AVID) Investigators. A comparison of anti-arrhythmic drug therapy with implantable defibrillators in patients resuscitated from near-fatal ventricular arrhythmias. N Engl J Med 1997;337:1576–83. https://doi.org/10.1056/NEJM199711273372202
- Theuns DAMJ, Smith T, Hunink MGM, Bardy GH, Jordaens L. Effectiveness of prophylactic implantation of cardioverter-defibrillators without cardiac-resynchronization therapy in patients with ischaemic or non-ischaemic heart disease: a systematic review and meta-analysis. Europace 2010;12:1564–70. https://doi.org/10.1093/europace/euq329
- Køber L, Thune JJ, Nielsen JC et al. Defibrillator implantation in patients with nonischaemic systolic heart failure. N Engl J Med 2016;375:1221–30. https://doi.org/10.1056/NEJMoa1608029 [Epub ahead of print]
- Moss AJ, Zereba W, Hall WJ et al. Prophylactic implantation of a defibrillator in patients with myocardial infarction and reduced ejection fraction. N Engl J Med 2002;346:877–83. https://doi.org/10.1056/NEJMoa013474 [Epub ahead of print]
- Rickard J, Wilkoff BL. Advances in implantable cardioverter defibrillator therapy. Expert Rev Cardiovasc Ther 2016;14:291–9. https://doi.org/10.1586/14779072.2016.1131124
- Bardy GH, Lee KL, Mark DB et al. Amiodarone or an implantable cardioverter-defibrillator for congestive heart failure. N Engl J Med 2005;352:225–37. https://doi.org/10.1056/NEJMoa043399
- Shen L, Jhund PS, Petrie MC et al. Declining risk of sudden death in heart failure. N Engl J Med 2017;377:41–51. https://doi.org/10.1056/NEJMoa1609758
- Daubert JP, Zareba W, Cannom DS et al. Implantable cardioverter-defibrillator shocks in MADIT II: frequency, mechanisms, predictors, and survival impact. J Am Coll Cardiol 2008;51:1357–65. https://doi.org/10.1016/j.jacc.2007.09.073
- Moss AJ, Schuger C, Beck CA et al. Reduction in inappropriate therapy and mortality through ICD programming. N Engl J Med 2012;367:2275–83. https://doi.org/10.1056/NEJMoa1211107 [Epub ahead of print]
- Lin G, Nishimura RA, Gersh BJ et al. Device complications and inappropriate implantable cardioverter defibrillator shocks in patients with hypertrophic cardiomyopathy. Heart 2009;95:709–14. https://doi.org/10.1136/hrt.2008.150656
- Ezzat VA, Lee V, Ahsan S et al. A systematic review of ICD complications in randomised controlled trials versus registries: is our ‘real-world’ data an underestimation? Open Heart 2015;2:e000198. https://doi.org/10.1136/openhrt-2014-000198
- McMurray JJV, Solomon SD, Inzucchi SE et al; DAPA-HF Trial Committees and Investigators. Dapagliflozin in patients with heart failure and reduced ejection fraction. N Engl J Med 2019;381:1995–2008. https://doi.org/10.1056/NEJMoa1911303 [Epub ahead of print]
- GOV.UK. Guidance: Cardiovascular disorders: assessing fitness to drive. 2024. https://www.gov.uk/guidance/cardiovascular-disorders-assessing-fitness-to-drive#implantable-cardioverter-defibrillator-icd (accessed 30 January 2024)
- Abraham WT, Adamson PB, Bourge RC et al; CHAMPION Trial Study Group. Wireless pulmonary artery haemodynamic monitoring in chronic heart failure: a randomised controlled trial. Lancet 2011;377:658–66. https://doi.org/10.1016/S0140-6736(11)60101-3
- Abraham WT, Stevenson LW, Bourge RC et al; CHAMPION Trial Study Group. Sustained efficacy of pulmonary artery pressure to guide adjustment of chronic heart failure therapy: complete follow-up results from the CHAMPION randomised trial. Lancet 2016;387:453-61. https://doi.org/10.1016/S0140-6736(15)00723-0 [Epub ahead of print]
- Lindenfeld JA, Zile MR, Desai AS et al. Haemodynamic-guided management of heart failure (GUIDE-HF): a randomised controlled trial. Lancet 2021;398:991–1001. https://doi.org/10.1016/S0140-6736(21)01754-2 [Epub ahead of print]
- Gardner RS, Singh JP, Stancak B et al. HeartLogic Multisensor Algorithm identifies patients during periods of significantly increased risk of heart failure events: results from the MultiSENSE study. Circ Heart Fail 2018;11:e004669. https://doi.org/10.1161/CIRCHEARTFAILURE.117.004669
- Ritzema J, Troughton R, Melton IT et al; HOMEOSTASIS Study Group. Physician-directed patient self-management of left atrial pressure in advanced chronic heart failure. Circulation 2010;121:1086–95. https://doi.org/10.1161/CIRCULATIONAHA.108.800490 [Epub ahead of print]
- Maurer MS, Adamson PB, Costanzo MR et al. Rationale and design of the left atrial pressure monitoring to optimize heart failure therapy study (LAPTOP-HF). J Card Fail 2015;21:479–88. https://doi.org/10.1016/j.cardfail.2015.04.012 [Epub ahead of print]
- Borggrefe MM, Lawo T, Butter C et al. Randomized, double-blind study of non-excitatory, cardiac contractility modulation electrical impulses for symptomatic heart failure. Eur Heart J 2008;29:1019–28. https://doi.org/10.1093/eurheartj/ehn020 [Epub ahead of print]
- Kadish A, Nademanee K, Volosin K et al. A randomized controlled trial evaluating the safety and efficacy of cardiac contractility modulation in advanced heart failure. Am Heart J 2011;161:329–37.e1–2. https://doi.org/10.1016/j.ahj.2010.10.025
- Zipes DP, Neuzil P, Theres H et al; DEFEAT-HF Trial Investigators. Determining the feasibility of spinal cord neuromodulation for the treatment of chronic systolic heart failure: the DEFEAT-HF study. JACC Heart Fail 2016;4:129–36. https://doi.org/10.1016/j.jchf.2015.10.006 [Epub ahead of print]
- Abraham WT, Zile MR, Weaver FA et al. Baroreflex activation therapy for the treatment of heart failure with a reduced ejection fraction. JACC Heart Fail 2015;3:487–96. https://doi.org/10.1016/j.jchf.2015.02.006 [Epub ahead of print]
- Gold MR, Van Veldhuisen DJ, Hauptman PJ et al. Vagus nerve stimulation for the treatment of heart failure: the INOVATE-HF Trial. J Am Coll Cardiol 2016;68:149–58. https://doi.org/10.1016/j.jacc.2016.03.525 [Epub ahead of print]
- McMurray JJV, Køber LV. End of the road for vagus nerve stimulation? J Am Coll Cardiol 2016;68:159–60. https://doi.org/10.1016/j.jacc.2016.05.025
- Corra U, Pistono M, Mezzani A et al. Sleep and exertional periodic breathing in chronic heart failure: prognostic importance and interdependence. Circulation 2006;113:44–50. https://doi.org/10.1161/CIRCULATIONAHA.105.543173 [Epub ahead of print]
- O’Connor CM, Whellan DJ, Fiuzat M et al. Cardiovascular outcomes with minute ventilation-targeted adaptive servo-ventilation therapy in heart failure: the CAT-HF Trial. J Am Coll Cardiol 2017;69:1577–87. https://doi.org/10.1016/j.jacc.2017.01.041
- Cowie MR, Woehrle H, Wegscheider K et al. Adaptive servo-ventilation for central sleep apnea in systolic heart failure (SERVE-HF). N Engl J Med 2015;373:1095–105. https://doi.org/10.1056/NEJMoa1506459 [Epub ahead of print]
- Silverstein ME, Ford CA, Lysaght MJ, Henderson LW. Treatment of severe fluid overload by ultrafiltration. N Engl J Med 1974;291:747–51. https://doi.org/10.1056/NEJM197410102911501
- Schroeder KL, Sallustio JE, Ross EA. Continuous haematocrit monitoring during intradialytic hypotension: precipitous decline in plasma refill rates. Nephrol Dial Transplant 2004;19:652–6. https://doi.org/10.1093/ndt/gfg590
- Marenzi G, Lauri G, Grazi M, Assanelli E, Campodonico J, Agostoni P. Circulatory response to fluid overload removal by extracorporeal ultrafiltration in refractory congestive heart failure. J Am Coll Cardiol 2001;38:963–8. https://doi.org/10.1016/s0735-1097(01)01479-6
- Marenzi G, Grazi S, Giraldi F et al. Interrelation of humoral factors, hemodynamics, and fluid and salt metabolism in congestive heart failure: effects of extracorporeal ultrafiltration. Am J Med 1993;94:49–56. https://doi.org/10.1016/0002-9343(93)90119-a
- Ali SS, Olinger CC, Sobotka PA et al. Enhanced sodium extraction with ultrafiltration compared to intravenous diuretics. J Card Fail 2006;12:S114. https://doi.org/10.1016/j.cardfail.2006.06.393
- Costanzo MR, Guglin ME, Saltzberg MT et al. Ultrafiltration versus intravenous diuretics for patients hospitalized for acute decompensated heart failure. J Am Coll Cardiol 2007;49:675–83. https://doi.org/10.1016/j.jacc.2006.07.073 [Epub ahead of print]
- Bart BA, Goldsmith SR, Lee KL et al. Ultrafiltration in decompensated heart failure with cardiorenal syndrome. N Engl J Med 2012;367:2296–304. https://doi.org/10.1056/NEJMoa1210357
- Costanzo MR, Negoianu D, Jaski BE et al. Aquapheresis versus intravenous diuretics and hospitalizations for heart failure (AVOID-HF trial). JACC Heart Fail 2016;4:95–105. https://doi.org/10.1016/j.jchf.2015.08.005 [Epub ahead of print]
- Brisco MA, Zile MR, Hanberg JS et al. Relevance of changes in serum creatinine during a heart failure trial of decongestive strategies: insights from the DOSE Trial. J Card Fail 2016;22:753–60. https://doi.org/10.1016/j.cardfail.2016.06.423 [Epub ahead of print]
- Kazory A, Bellamy FB, Ross EA. Ultrafiltration for acute decompensated heart failure: financial implications. Int J Cardiol 2012;154:246–9. https://doi.org/10.1016/j.ijcard.2011.05.073 [Epub ahead of print]
- Clark AL, Gardner RS, McDonagh TA (eds.). Oxford Textbook of Heart Failure (2nd edn.). Oxford: Oxford University Press, 2022:1–896.
- National Human Genome Research Institute. Gene Therapy. NIH, 2024. Available at: https://www.genome.gov/genetics-glossary/Gene-Therapy (accessed 30 January 2024)
- Hulot JS, Ishikawa K, Hajjar RJ. Gene therapy for the treatment of heart failure: promise postponed. Eur Heart J 2016;37:1651–8. https://doi.org/10.1093/eurheartj/ehw019 [Epub ahead of print]
- Nattel S, Maguy A, Le Bouter S, Yeh YH. Arrhythmogenic ion-channel remodeling in the heart: heart failure, myocardial infarction, and atrial fibrillation. Physiol Rev 2007;87:425–56. https://doi.org/10.1152/physrev.00014.2006
- Hulot J-S, Salem JE, Redheuil A et al; AGENT-HF Investigators. Effect of intracoronary administration of AAV1/SERCA2a on ventricular remodelling in patients with advanced systolic heart failure: results from the AGENT-HF randomized phase 2 trial. Eur J Heart Fail 2017;19:1534−41. https://doi.org/10.1002/ejhf.826 [Epub ahead of print]
- Greenberg B, Butler J, Felker GM et al. Calcium upregulation by percutaneous administration of gene therapy in patients with cardiac disease (CUPID 2): a randomised, multinational, double-blind, placebo-controlled, phase 2b trial. Lancet 2016;387:1178–86. https://doi.org/10.1016/S0140-6736(16)00082-9 [Epub ahead of print]
- Gabisonia K, Recchia FA. Gene therapy for heart failure: new perspectives. Curr Heart Fail Rep 2018;15:340−9. https://doi.org/10.1007/s11897-018-0410-z
- Emdin M, Aimo A, Rapezzi C et al. Treatment of cardiac transthyretin amyloidosis: an update. Eur Heart J 2019;40:3699–706. https://doi.org/10.1093/eurheartj/ehz298
- Benson MD, Waddington-Cruz M, Berk JL et al. Inotersen treatment for patients with hereditary transthyretin amyloidosis. N Engl J Med 2018;379:22−31. https://doi.org/10.1056/NEJMoa1716793
- Adams D, Gonzalez-Duarte A, O’Riordan WD et al. Patisiran, an RNAi therapeutic, for hereditary transthyretin amyloidosis. N Engl J Med 2018;379:11−21. https://doi.org/10.1056/NEJMoa1716153
- Sperry BW, Tang WHW. Amyloid heart disease: genetics translated into disease-modifying therapy. Heart 2017;103:812–7. https://doi.org/10.1136/heartjnl-2016-309914
- Adams D, Gonzalez-Duarte A, O’Riordan WD et al. Patisiran, an RNAi therapeutic, for hereditary transthyretin amyloidosis. N Engl J Med 2018;379:11–21. https://doi.org/10.1056/NEJMoa1716153
- Gao J, Dennis JE, Muzic RF, Lundberg M, Caplan AI. The dynamic in vivo distribution of bone marrow-derived mesenchymal stem cells after infusion. Cells Tissues Organs 2001;169:12–20. https://doi.org/10.1159/000047856
- Chong JJ, Murry CE. Cardiac regeneration using pluripotent stem cells–progression to large animal models. Stem Cell Res 2014;13:654–65. https://doi.org/10.1016/j.scr.2014.06.005 [Epub ahead of print]
- National Institute for Health and Care Excellence. Acute heart failure: diagnosis and management. NICE clinical guidance [CG187]. London: NICE, 2021. Available from: https://www.nice.org.uk/guidance/cg187 (accessed October 2023)
- Glikson M, Nielsen JC, Kronborg MB et al; ESC Scientific Document Group. 2021 ESC Guidelines on cardiac pacing and cardiac resynchronization therapy: Developed by the Task Force on cardiac pacing and cardiac resynchronization therapy of the European Society of Cardiology (ESC) With the special contribution of the European Heart Rhythm Association (EHRA). Eur Heart J 2021;42:3427–520. https://doi.org/10.1093/eurheartj/ehab364
All rights reserved. No part of this programme may be reproduced, stored in a retrieval system, or transmitted in any form or by any means, electronic, mechanical, photocopying, recording or otherwise, without the prior permission of the publishers, Medinews (Cardiology) Limited.
It shall not, by way of trade or otherwise, be lent, re-sold, hired or otherwise circulated without the publisher’s prior consent.
Medical knowledge is constantly changing. As new information becomes available, changes in treatment, procedures, equipment and the use of drugs becomes necessary. The editors/authors/contributors and the publishers have taken care to ensure that the information given in this text is accurate and up to date. Readers are strongly advised to confirm that the information, especially with regard to drug usage, complies with the latest legislation and standards of practice.
Healthcare professionals should consult up-to-date Prescribing Information and the full Summary of Product Characteristics available from the manufacturers before prescribing any product. Medinews (Cardiology) Limited cannot accept responsibility for any errors in prescribing which may occur.